The Nervous System includes the Central Nervous System (CNS), consisting of the brain and the spinal cord, and the Peripheral Nervous System (PNS), consisting of ganglia and cranial and spinal nerves. The nervous system is composed of nervous tissue, whose function is to receive, process and transmit impulses.
The functional unit of this tissue is a highly specialized cell with a precise structure. It is excitable and has the ability to transmit impulses: it is called neuron. Other cell types, collectively known as neuroglia, or simply glia, aid them. The neuron-neuroglial cells ratio is approximately 1:1. In an adult individual brain there are, on average, 86.1± 8.1 billion neurons and 84.6 ± 9.8 neuroglial cells (Azevedo FAC, Carvalho LRB, Grinberg LT, Farfel JM, Ferretti RE, Leite RE, Jacob Filho W, Lent R, Herculano-Houzel SJ. Equal numbers of neuronal and non-neuronal cells make the human brain an isometrically scaled-up primate brain. Comp Neurol. 2009 513(5):532-41, doi: 10.1002/cne.21974).
The neuron
Each neuron consists of a cell body, called soma, which includes the nucleus and cytoplasm (perikaryon) from which one or more cytoplasmic processes leave. Such processes are suitable to receive impulses and they are known as dendrites. The other neuronal component is the only cytoplasmic protrusion that transmits impulses: the axon (also known as neurite). The nucleus is located inside the soma, together with the cytoplasmic organelles and several ribosomes associated with the rough endoplasmic reticulum to form clusters. Such clusters are known as tigroid substance (Nissl bodies) and can be highlighted as basophilic granules with a light microscope. Dendrites are thin and highly branched. They can also present protrusions called spines. Generally, axons are much longer than dendrites. They arises from the soma, in the axon hillock, and can repeatedly branch out. Each branch ends with a button-shaped swelling: the synaptic ending or bouton. Synaptic endings can connect with both neurons and effector organs. In the former case, synaptic endings are classified according to the neural region they connect with. Therefore, synaptic endings can be: axosomatic, axodendritic, axospinous and axoaxonic.
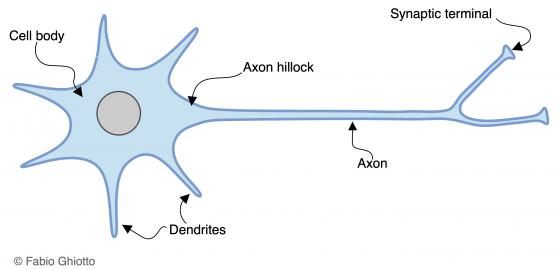
Figure N1. Schematic drawing of the neuron architecture.

Figure N2. Schematic drawing of the different synapsing modalities between neurons.
Depending on the number of dendrites, neurons can be divided into:
- unipolar neurons: only present during embryonic life. They have no dendrites and their single, central extension operates as an axon;
- pseudounipolar neurons: their soma has a drop shape. They have only one extension that later splits and takes on a T-shape, giving rise to an axon and a dendrite. Some examples are the neurons of spinal sensory ganglia and of cranial nerve ganglia;
- bipolar neurons: their soma has an elliptical shape with two extensions, the axon and the dendrite, located at opposite poles of the cell. They can be found in the spiral ganglion of Corti, vestibular ganglion, olfactory epithelium and retina;
- multipolar neurons: they have a polyhedral shape since one axon and two or more dendrites leave from the soma. They represent the most common type of neuron in the nervous tissue.
The neuroglial cells
In contrast to neurons, these cells generally maintain their proliferative capacity. Their aim is to support and cooperate during the neuronal functions. In the CNS, there are oligodendrocytes, astrocytes, microglia and ependymal cells, while in the PNS there are the Schwann cells and satellite cells.
Oligodendrocytes and Schwann cells: the myelination process of fibers
Oligodendrocytes and Schwann cells form the myelin in the CNS and PNS, respectively. The myelin is a lining formed by the wrapped oligodendrocyte/Schwann cell membrane, surrounding a section of the axon. Multiple following sections of the lining cover the whole axon with myelin. Therefore, there are various types of fibers.
- Myelinated fibers: they are present both in the CNS and in the PNS. In the CNS, every olygodendrocyte can wrap one section of different axons, while in the PNS every section of the axon is enveloped by a single Schwann cell. Every Schwann cell lines a single axon section, and so every axon is lined by more Schwann cells one behind the other. The spaces between the Schwann cells are called nodes of Ranvier and they give rise to a saltatory impulse conduction. The same myelin arrangement can be found in the CNS (oligodendrocytes).
- Unmyelinated fibers: in these fibers, axons are not enveloped by a myelinic sheath. In this case, several axons are immersed in Schwann cell cytoplasm, surrounded by its plasma membrane. In humans, this type of fibers is especially present in the Visceral Nervous System (Autonomic Nervous System).
- Naked axons: they present no lining. In the CNS fibers without a myelin coat are naked axons. Naked axons can also be found in free nerve endings.If nerve fibres are not stained with a selective dye, they appear to be poorly stained and with a highly emphasized typical sinusoidal trend under a light microscope.
Astrocytes
Astrocytes can be found in the CNS, where they represent the most important physical support for neurons and they contribute to creating the blood-brain barrier. They have a star-like shape and their extensions end with pedicels. Astrocytes are divided into two categories: fibrous astrocytes, which are more common in the white matter and present few long cytoplasmic extensions, and protoplasmic astrocytes, which are more common in the gray matter and present short-branched cytoplasmic extensions.
Microglia cells
These cells derive from the mesoderm and have a main phagocytic function. This is why they are considered the effectors of the immune protection of the CNS.
Ependymal cells
They line the brain ventricles, the central canal and the choroid plexuses.
In the first two cases, we have a cylindrical epithelium lining the cavities of the brain ventricles and the central canal of the spinal cord. In contrast to other types of cells, ependymal cells do not lay on a basement membrane and are not tightly joined. Instead, they present thin branching crossing with those of the underlying astrocytes. They present cilia on their apical surface. In the third case, we have structurally modified ependymal cells, specialized in secreting functions, which line the choroid plexuses. These cells, in contrast to the first ones, lie on a basement membrane, are bound to each other by tight junctions, and display multiple microvilli on their apical surface. They contribute to the production of the cerebrospinal fluid.
Ganglia
These are aggregations of neuronal cell bodies which can be found along the peripheral nervous fibers. They may present a connective capsule.
They are classified into:
- Spinal sensory ganglia and cranial nerve ganglia. Spinal sensory ganglia are ovoid, quite large and lined by a thick connective capsule. They are located on the sides of the spinal cord, in the posterior root of the spinal nerve. Internally, there are cell bodies of pseudounipolar neurons. These neurons are mostly located in peripheral areas, since the center of the ganglion is occupied by a dense tangle of nerve fibers leaving from the pseudounipolar neurons themselves. The cell bodies of pseudounipolar neurons are almost completely surrounded by satellite cells, connected to the reticular fibers coming from the connective capsule. These satellite cells provide neurons with support. Sensory ganglia of the cranial nerves are formed by pseudounipolar neurons surrounded by satellite cells too.
- Ganglia of the visceral nervous system (both sympathetic and parasympathetic): they are generally smaller than spinal sensory ganglia and they are formed by multipolar neurons uniformly mixed with fibers, so that there are no morphologically and topographically distinguishable zones. Because the neurons of the visceral ganglia are multipolar, their cell bodies are not completely surrounded by satellite cells. In fact, the latter are scarce and hardly visible. In several cases, parasympathetic ganglia are formed by few neurons, they have no connective capsule and they are found inside other organs. In this case, they are called “intramural ganglia”.
Receptor system
The human body owns receptor systems that allow it to receive information on the surrounding environment. Such senses can be either special (such as vision, hearing, balance, taste and smell) or general, collecting various types of information (e.g. thermal and tactile). The receptors constituting these systems are divided into exteroceptors, proprioceptor and enteroceptors. This classification depends on where they collect the information from: from an external environment (exteroceptors), from muscles, tendons and ligaments (proprioceptors) or from viscera (enteroceptors). Another more recent receptor classification is based on the type of stimulus needed to activate them (called adequate stimulus). This classification distinguishes receptors in chemoreceptors, photoreceptors, thermoreceptors and mechanoreceptors. There is one more group of receptors with particular features, regarding both the adequate stimulus and the response to the stimulus itself. This is the group of pain receptors, called nociceptors.
Somatosensory receptors include free nerve endings and encapsulated nerve endings.
- Free nerve endings are formed by thin fibers that, having lost their myelin sheath, are scattered around the epithelial tissue or connective tissue of multiple organs. On their top, they present bouton-like expansions that receive stimuli of different types.
- Encapsulated nerve endings are surrounded by mature connective tissue and their morphology varies remarkably. The most common are Pacinian corpuscles, Meissner’s corpuscles and Ruffini’scorpuscles. Their arrangement allows for the association between the free nerve ending and a system of connective lamellae, mostly concentric, that filter and/or amplify the adequate stimulus.
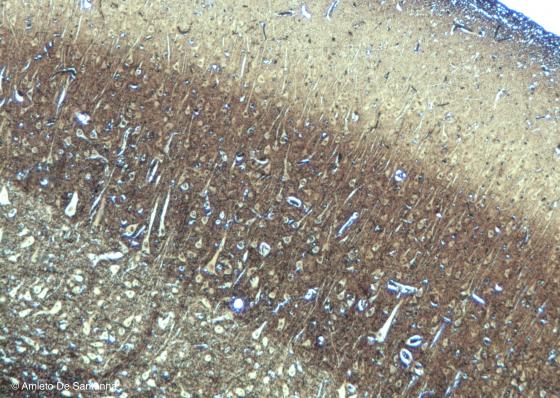
Figure N3. Pig telencephalon. Pyramidal neurons of the cerebral cortex. Golgi-Cox X63
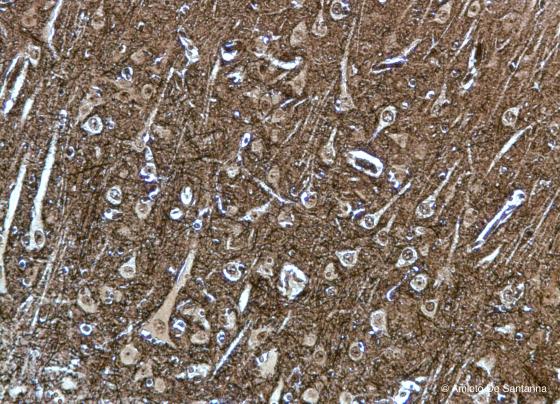
Figure N4. Pig telencephalon at higher magnification. Pyramidal cells and few nerve fibers are clearly visible. Golgi-Cox X100
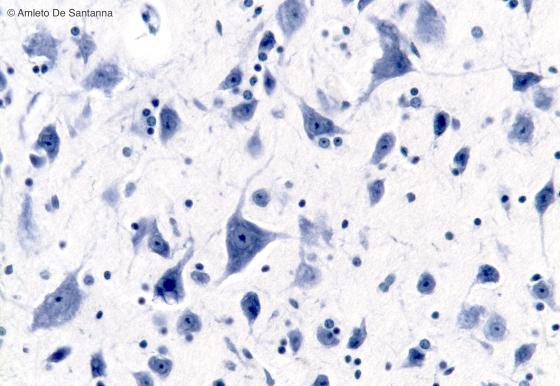
Figure N5. Human brainstem. Multipolar neurons in the medulla oblongata. In the neuron, cell bodies are clearly visible Nissl bodies. In the neuron nuclei, when the cutting plane is appropriate, the nucleoli are clearly visible regardless the staining method used. The roundish smaller nuclei are glial cell nuclei. Nissl X200.
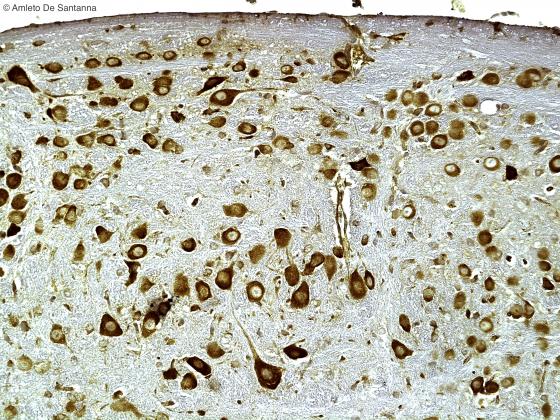
Figure N6. Mouse cerebral cortex. Pyramidal neurons at high magnification. The apical dendrite is characteristic of these nerve cells. DAB X100
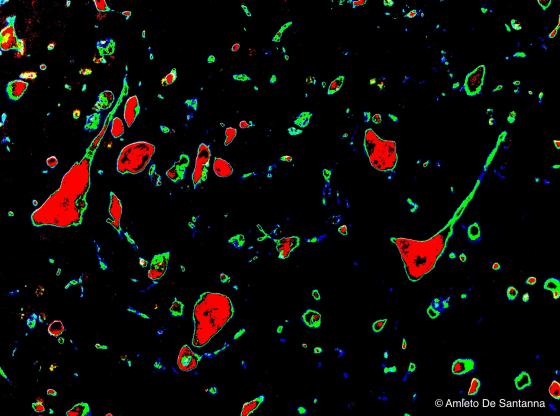
Figure N7. Mouse cerebral cortex. Digitally processed micrograph that shows pyramidal neurons: the cell soma are in red, dendrites, fibers and glial cells in green. X200.
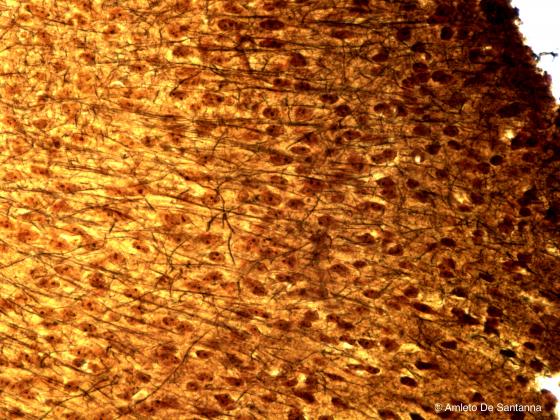
Figure N8. Human cerebral cortex. Black stained nervous fibers are visible on a brownish background. Golgi X400
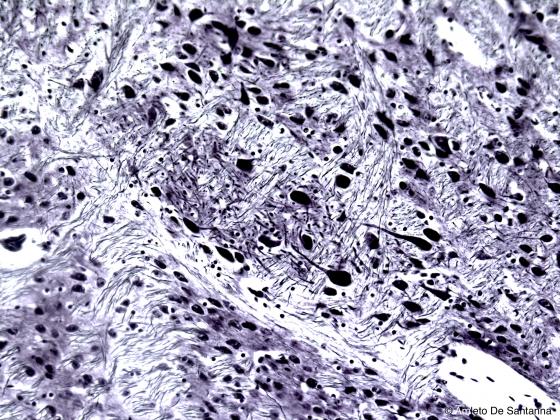
Figure N9. Human cerebral cortex. Nervous fibers and neurons are clearly visible stained black. Bielschowsky X200
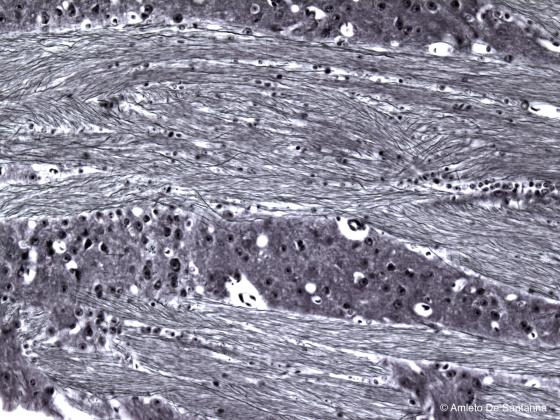
Figure N10. Human brain. The nerve fibers are clearly visible. Bielschowsky X200
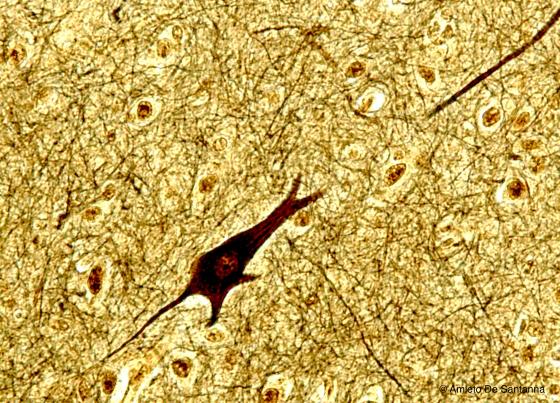
Figure N11. Human brain. Multipolar neuron of the cerebral cortex. Cajal X200
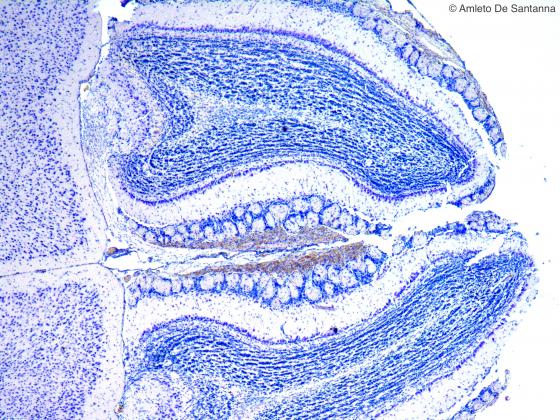
Figure N12. Mouse olfactory bulb at low magnification. Nissl X40
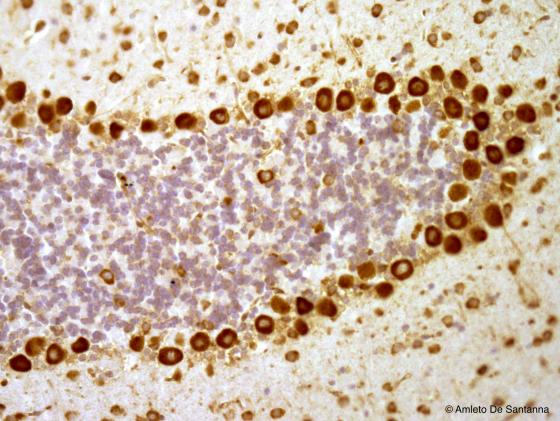
Figure N13. Mouse cerebellum. This micrograph clearly shows the three layered cytoarchitecture of the cerebellar cortex. This 10 µm thick section is not thick enough to show the Purkinje cell dendrites DAB X200
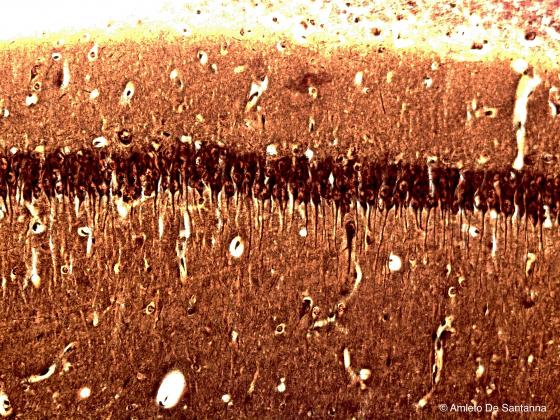
Figure N14. Mouse cerebellum. Digitally processed micrograph that shows the Purkinje cells in red. X200
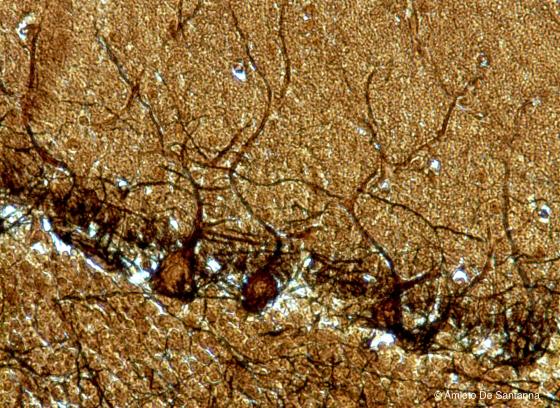
Figure N15. Human cerebellum. Purkinje cells at higher magnification. The section thickness of this specimen is greater (18 µm) to allow a better view of the Purkinje cell dendrites. Golgi X200
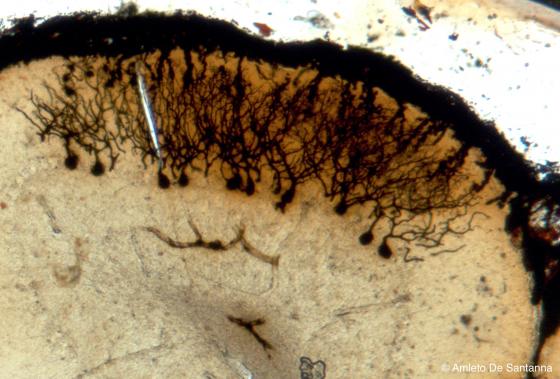
Figure N16. Mouse cerebellum. Sample obtained after staining and soaking of the tissue. The sample was obtained by squeezing a very thick section (200-300 µm). All the parts of the Purkinje cells are visible. Golgi-Cox X63
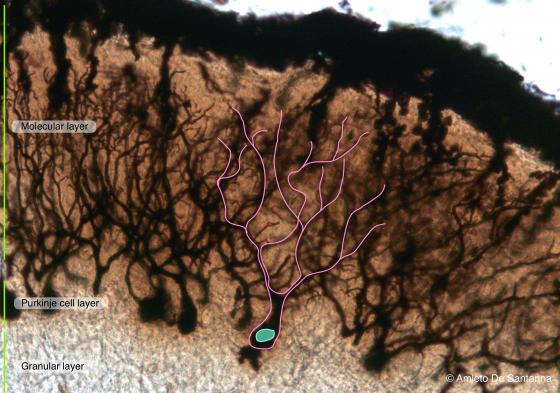
Figure N17. Mouse cerebellum at higher magnification. A Purkinje cell is outlined in pink. Golgi-Cox X100
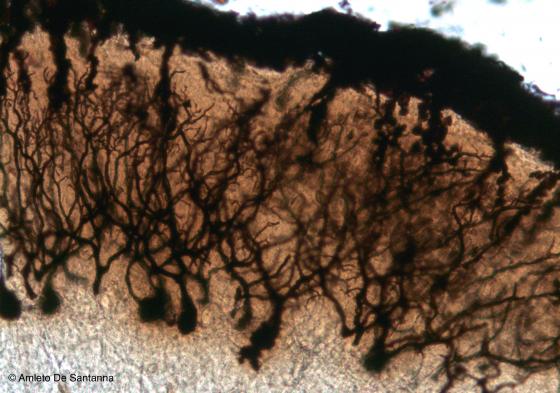
Figure N18. Mouse cerebellum at higher magnification. All the parts of the Purkinje cells are visible. Golgi-Cox X100
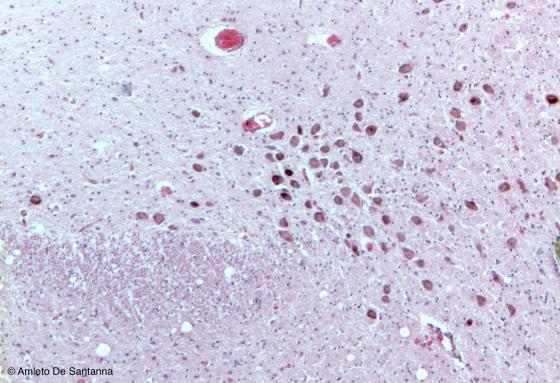
Figure N19. Mouse brainstem. Multipolar neurons that were cut on a transverse plane. The brown granulation, specific of these neurons, is due to intracellular melanin storage. H&E X63
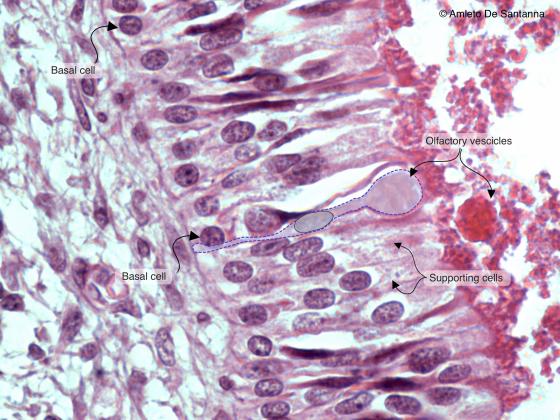
Figure N20A. Human fetal olfactory mucosa. You can see, at high magnification, a bipolar olfactory neuron (outlined) whose apical dendrite forms a knoblike ending. An axon originates at the base of the neuron (not shown) which will cross the ethmoid lamina cribrosa to reach the olfactory bulb inside the skull. All these axons form the olfactory nerve (cranial nerve I). H&E X630
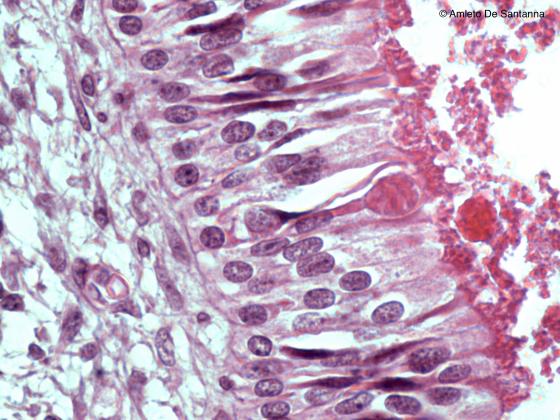
Figure N20B. Human fetal olfactory mucosa. In this micrograph, you can see a bipolar olfactory neuron whose apical dendrite forms a knoblike ending that is called olfactory vesicle. H&E X630
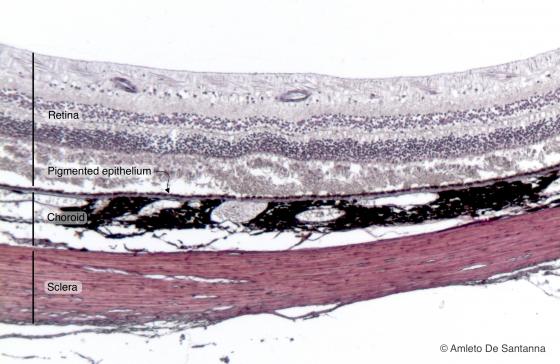
Figure N21A. Human eye. Retina, pigmented epithelium, choroid and sclera are easily seen. H&E X40
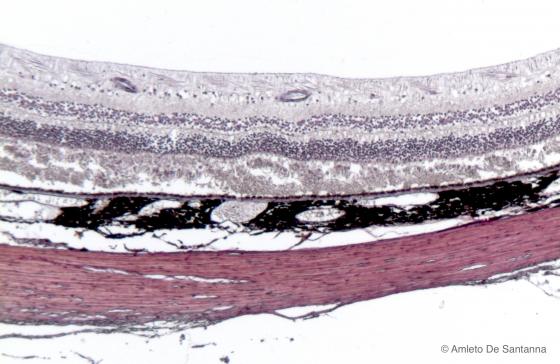
Figure N21B. Human eye. Retina, pigmented epithelium, choroid and sclera are easily seen. H&E X40
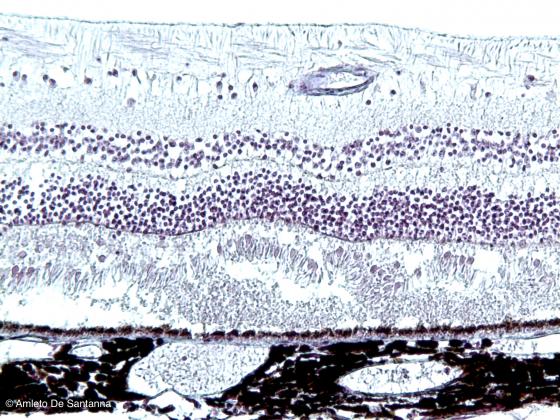
Figure N22. Human eye. Neural coat and choroid. In the neural coat, the pigmented layer and the retina, with cones and rods, are identifiable. H&E X100
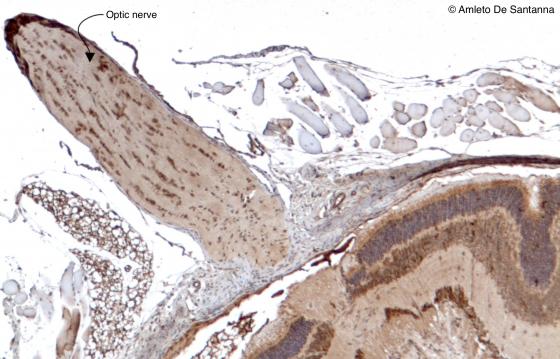
Figure N23. Mouse optic nerve. You can easily see the wavy shape of the fibers that form the optic nerve. HRP DAB X63
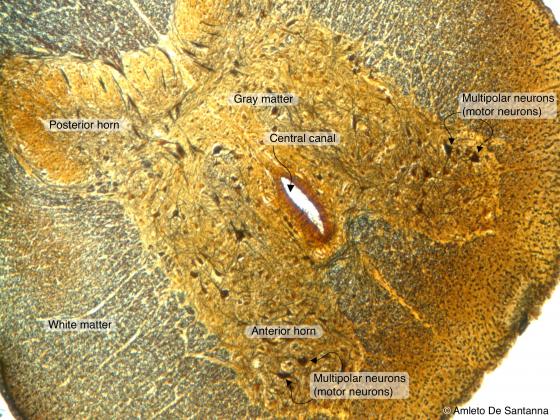
Figure N24A. Cat spinal cord. Transverse section. You can identify the central canal and the gray matter rich in multipolar neurons centrally surrounded by the nerve fibers making up the white matter. Golgi X63
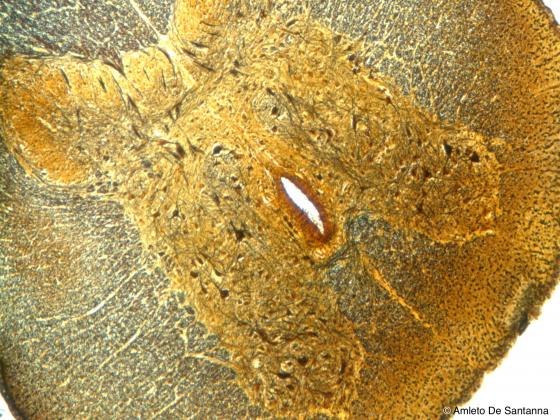
Figure N24B. Cat spinal cord. Transverse section. You can identify the central canal and the gray matter rich in multipolar neurons centrally surrounded by the nerve fibers making up the white matter. Golgi X63
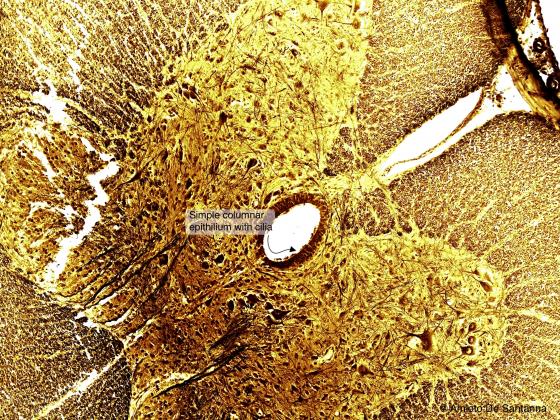
Figure N25. Cat spinal cord. Transverse section. In the middle, you can see the central canal lined by simple cylindrical epithelium. Of note, there is no basal membrane. Golgi X200
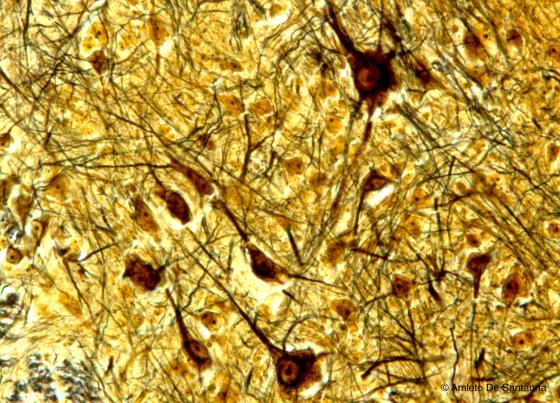
Figure N26. Cat spinal cord at higher magnification. You can see the motor neurons in the anterior horns of the spinal cord. Golgi X200
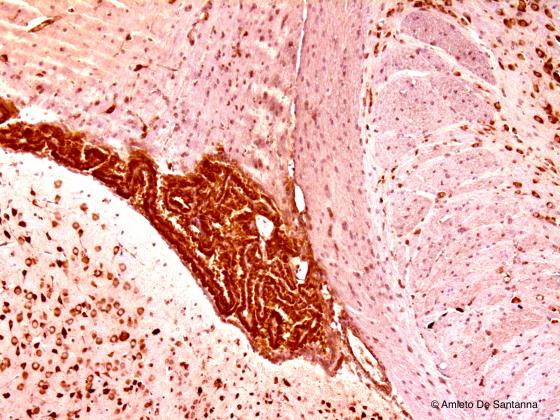
Figure N27. Mouse brain. Choroidal plexuses. DAB X40
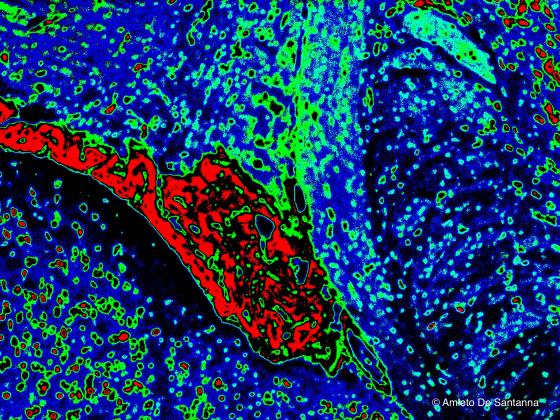
Figure N28. Mouse brain. Digitally processed micrograph. Choroidal plexuses are in red. X40
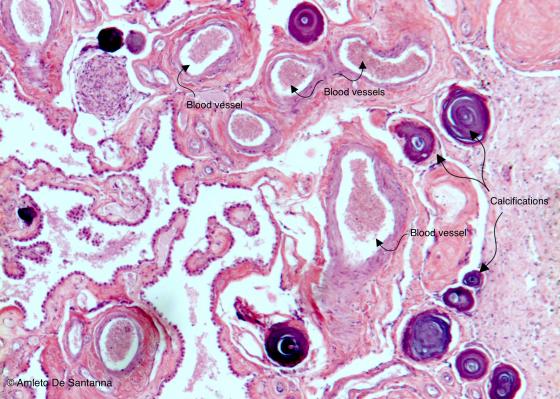
Figure N29. Human brain. Choroidal plexuses with several calcifications. As you can see, several blood vessels are present. H&E X25
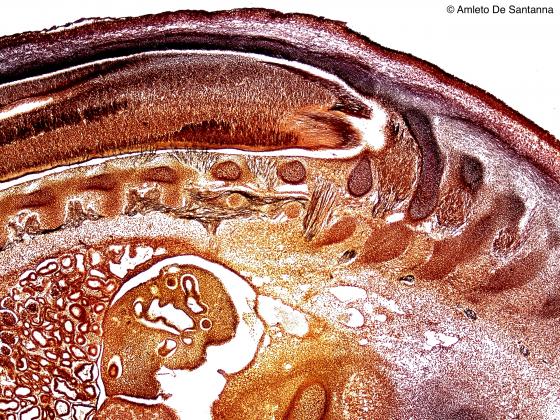
Figure N30. Human fetus. Longitudinal section showing the vertebral column, still partially ossified, and numerous, thin nerve fibers, stained in black, which enter small spinal sensory ganglia. Golgi-Cox X25
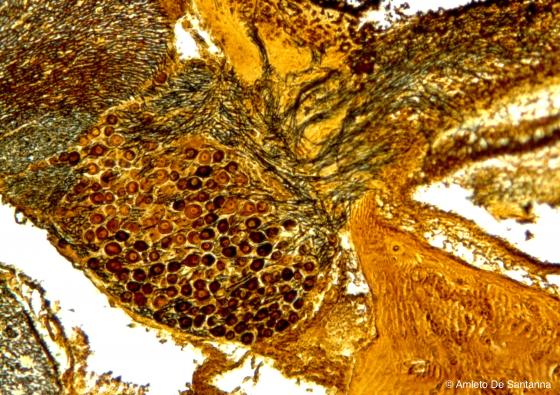
Figure N31. Human fetal dorsal root ganglion. Ganglion in its natural position. You can see the pseudounipolar neurons and the high number of nerve fibers that leave the ganglion forming the posterior root. Golgi-Cox X63
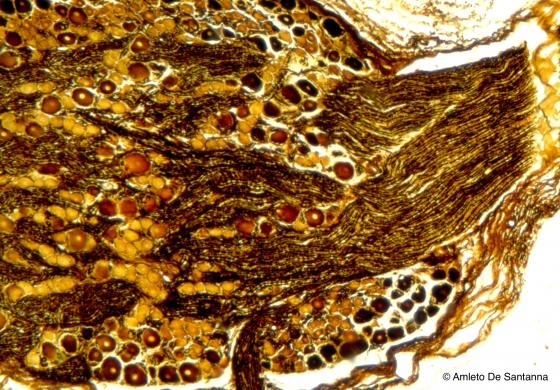
Figure N32. Human fetal dorsal root ganglion at higher magnification. A large bundle of fibers leaves the ganglion displacing the neuron bodies peripherally and enters the spinal cord. This is a morphological landmark that allows to distinguish a dorsal root ganglion from a visceral ganglion. In dorsal root ganglia, gangliar cells are grouped in islands separated by large fiber bundles or are placed peripherally. Golgi-Cox X100
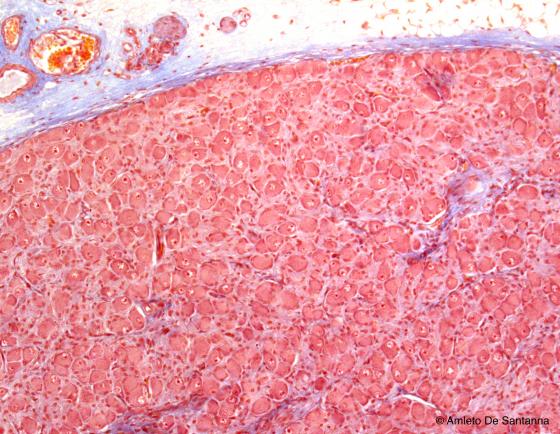
Figure N33. Human mesenteric ganglion. In this kind of ganglion, there are less fibers than the dorsal root ganglion and are regularly distributed throughout the ganglion itself as are the neurons. Azan-Mallory X63

Figure N34. Human mesenteric ganglion. In visceral ganglia, neurons and fibers are evenly distributed. Moreover, the neurons in these ganglia are multipolar and are incompletely surrounded by satellite cells. Azan-Mallory X200

Figure N35. Visceral ganglion at high magnification. You can see the low number of satellite cells (arrows) around multipolar neurons. H&E X200
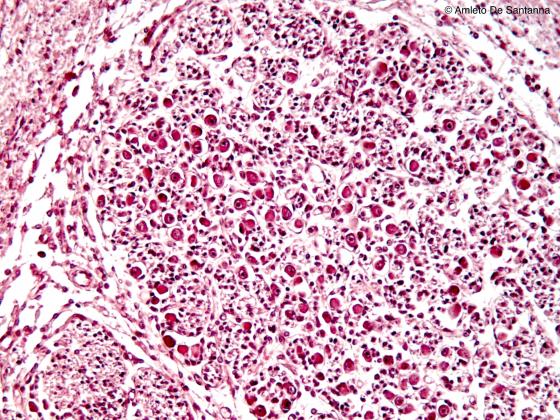
Figure N36. Human fetal internal ear. Cochlear ganglion. H&E X100
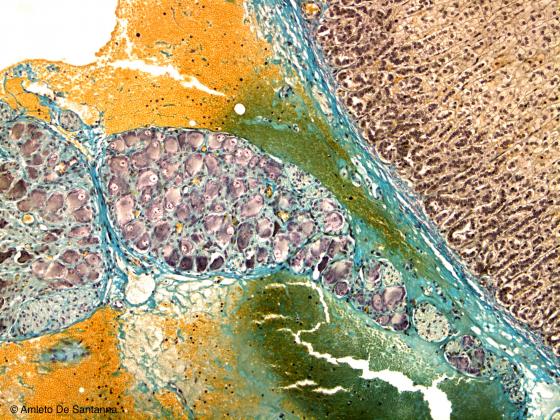
Figure N37. Rabbit visceral ganglion. Modified Mallory X100
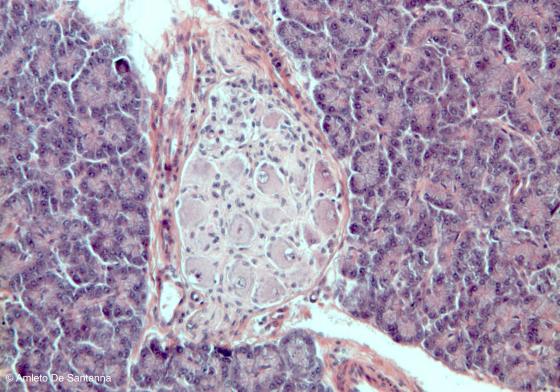
Figure N38. Mouse pancreas. Parasympathetic ganglion in the pancreas connective tissue. H&E X100
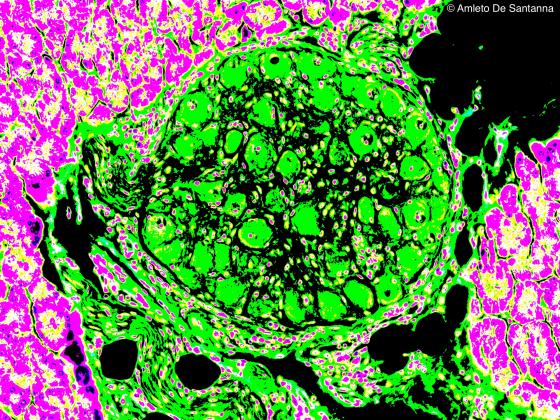
Figure N39. Mouse pancreas. Digitally processed micrograph. Small ganglion (green) of the parasympathetic nervous system present in the connective tissue of the pancreas. X160
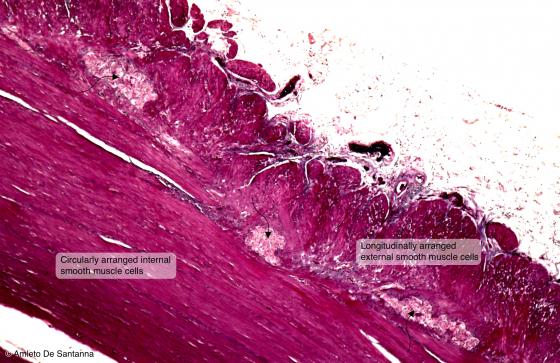
Figure N40. Human gut. Digitally processed micrograph. You can see the myenteric plexus (arrows). X63
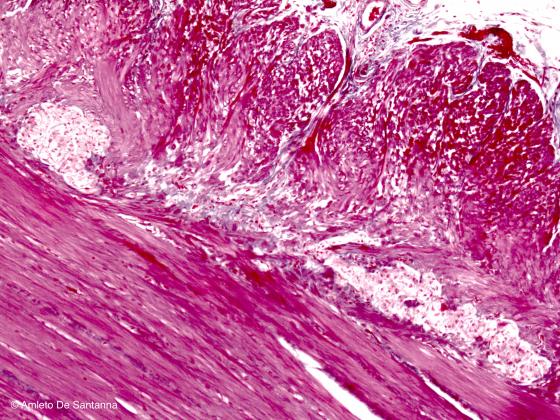
Figure N41. Human gut. Myenteric plexus at higher magnification. H&E X100
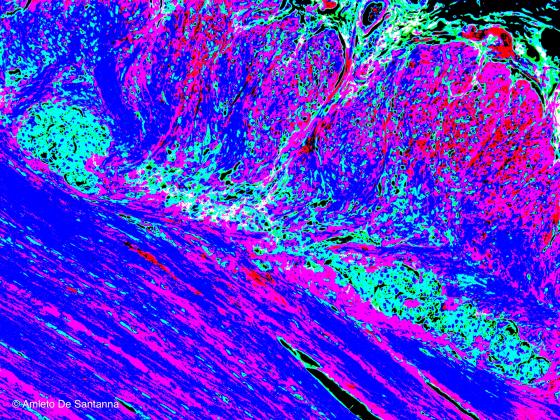
Figure N42. Human gut. Digitally processed micrograph. Myenteric plexus at higher magnification (light blue) X100
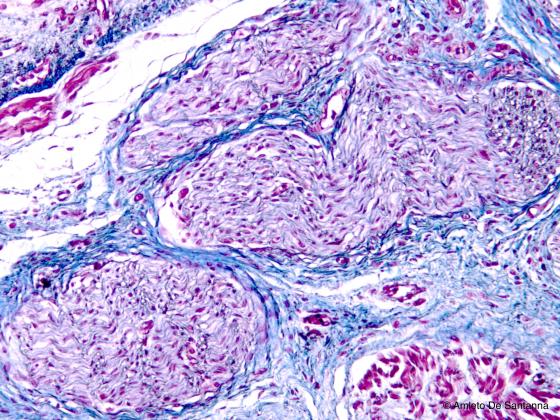
Figure N43. Human fetal tongue. Peripheral nerve at low magnification. Using conventional staining techniques, the peripheral nerve is always poorly stained. In this micrograph, you can identify a nerve fiber bundle with its typical wavy progression. Azan-Mallory X100
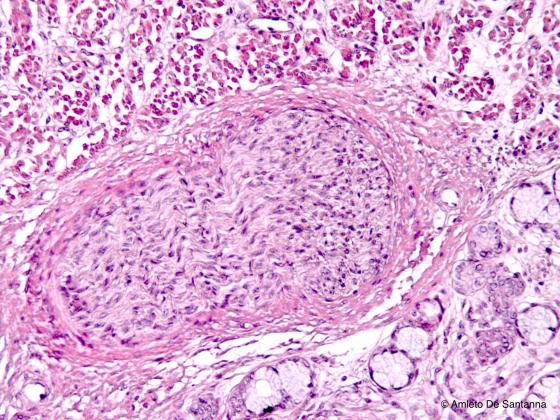
Figure N44. Human fetal tongue. Peripheral nerve. H&E X100
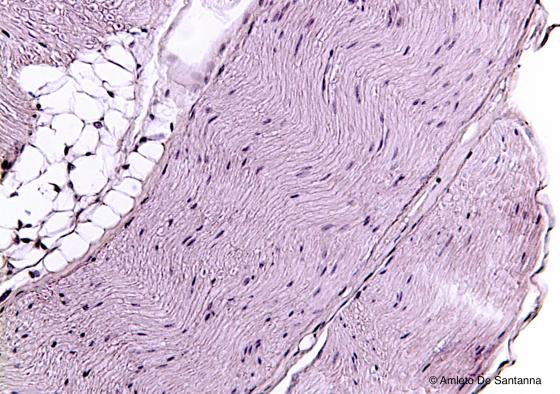
Figure N45. Mouse sciatic nerve, longitudinal section. The nerve fibers, usually poorly stained, have the typical wavy progression. The visible nuclei are the ones of the Schwann cells. H&E X100
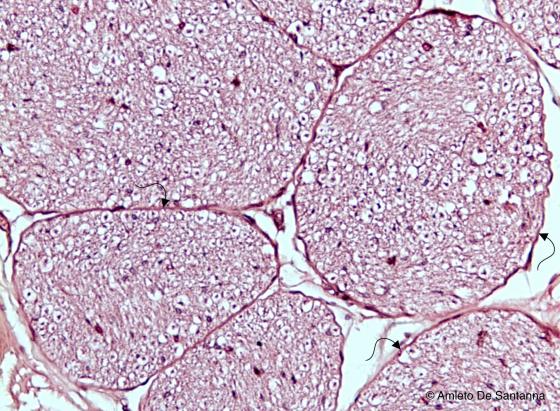
Figure N46. Human peripheral nerve. Nerve fiber bundles (funicula) are cut transversely and separated by a thin connective layer, the perinevrium (arrow). The empty spaces around the cells are artifacts due to the high amount of lipids of the myelin sheats. H&E X100
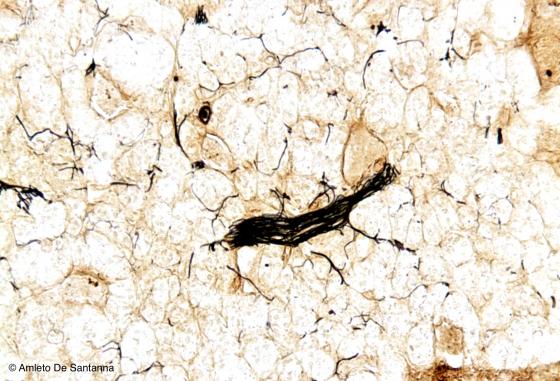
Figure N47. Human surrenal gland. You can see small fiber bundles and individual fibers of the surrenal gland medulla selectively stained black. Bielschowsky X100

Figure N48. Human fetal dorsal root ganglion. You can see nerve fibers, stained black, that leave the dorsal root ganglion. Golgi X100
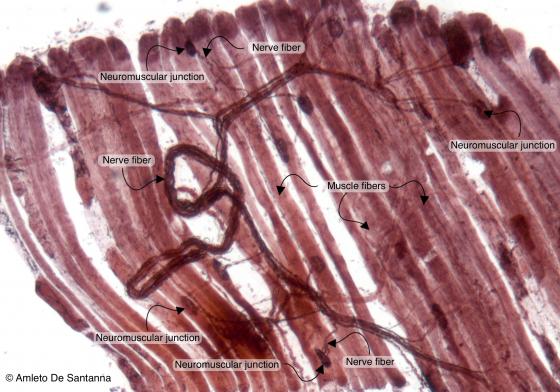
Figure N49A. Frog neuromuscular junctions. Selective staining for neuromuscular junctions. You can see a fiber bundle that ends with neuromuscular junctions allowing the triggering of muscular contraction. Ruffini X100
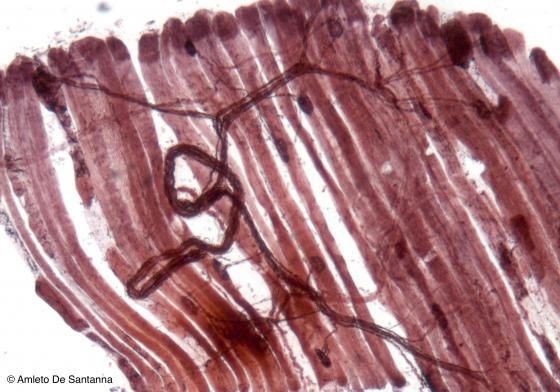
Figure N49B. Frog neuromuscular junctions. Selective staining for neuromuscular junctions. You can see a fiber bundle that ends with neuromuscular junctions allowing the triggering of muscular contraction. Ruffini X100
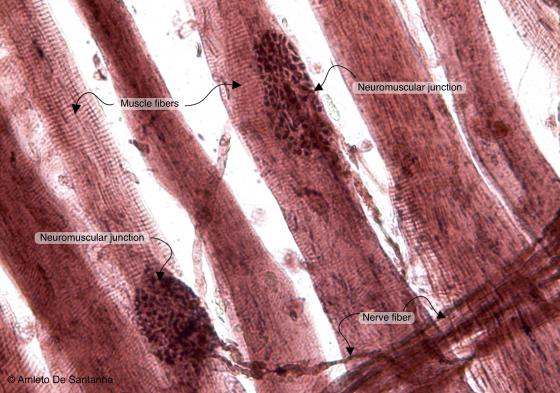
Figure N50A. Frog neuromuscular junctions at higher magnification. You can easily see the fiber ending (motor end plate) adjoining the skeletal muscle fiber. Ruffini X200
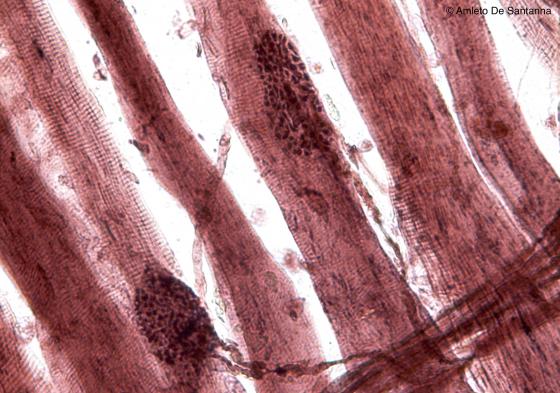
Figure N50B. Frog neuromuscular junctions at higher magnification. You can easily see the fiber ending (motor end plate) adjoining the skeletal muscle fiber. Ruffini X200
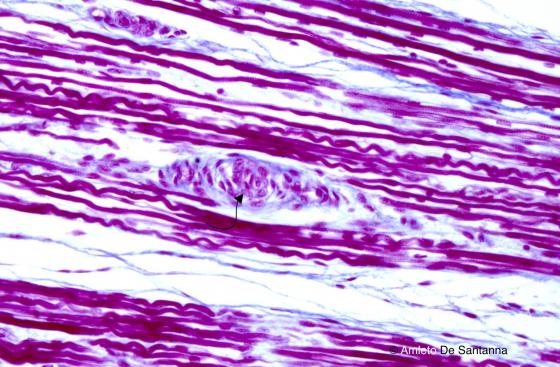
Figure N51. Fetal human tongue. Muscle spindle (arrow). Azan-Mallory X200
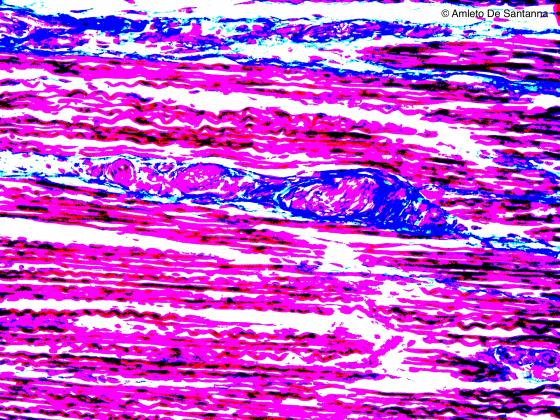
Figure N52. Fetal human tongue. Digitally processed micrograph. Muscle spindle. The connective tissue is evident (blue). X200
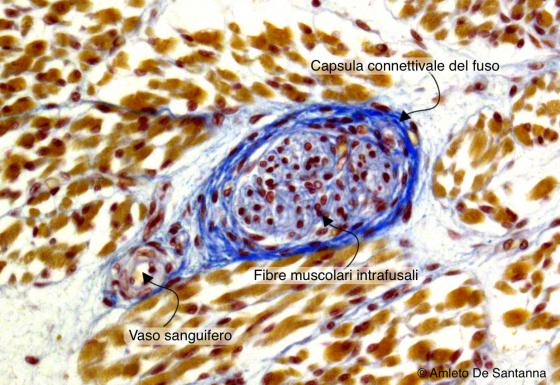
Figure N53. Human fetal tongue. Muscle spindle. Ignesti X300
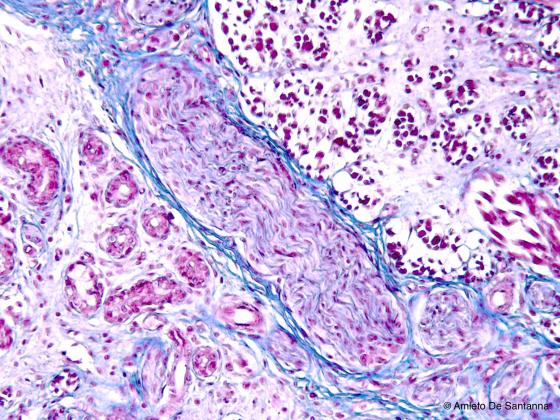
Figure N54. Human fetal tongue. Muscle spindle sectioned obliquely. Azan-Mallory X63
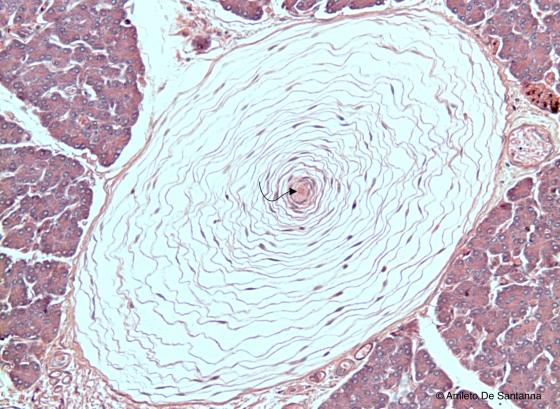
Figure N55. Pacinian corpuscles. Transverse section. The nerve ending (arrow) at the center of the corpuscle receive the vibratory input whose frequency is filtered by the lamellar complex that surrounds the fiber itself. H&E X200
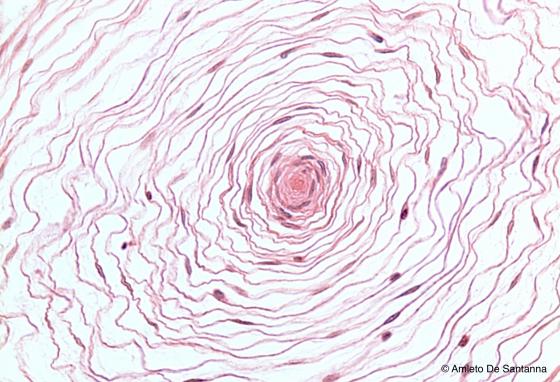
Figure N56. Pacinian corpuscle at higher magnification.You can see the nerve ending (center) and the large lamellar complex that surrounds it. H&E X200
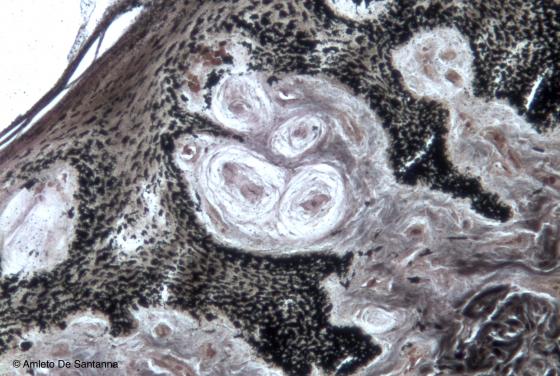
Figure N57. Whale skin. Groups of Pacinian corpuscles surrounded by capillary vessels. In marine mammals, the Pacinian corpuscle function is not tactile, it is rather that of signaling a pressure change. In this way, as the depth increases, the blood flow increases too, matching the animal blood pressure with the external pressure. This process allows a proper thermal regulation. Bielschowsky X100
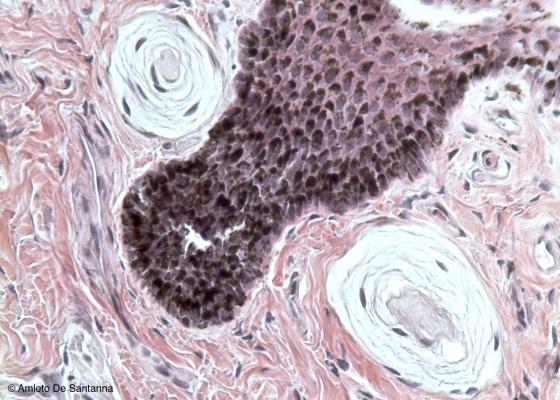
Figure N58. Whale skin. Two Pacinian corpuscles just beneath the epidermis. H&E X200