These tissues owe their name to their particular functions, which provide them with histological features clearly distinguishing them from other connective tissues.
They are:
- Adipose tissue
- Cartilage
- Bone tissue
- Lymphatic tissue
- Blood
Note: cartilage and bone tissue are commonly known as supporting connective tissue.
Adipose tissue
The cells composing adipose tissue are called adipocytes and their function is to accumulate fats into cytoplasmic vacuoles.
There are two types of adipose tissue: white (unilocular) and brown (multilocular)
White adipose tissue
Its adipocytes contain a single large drop of lipid (the reason why it is called unilocular). This drop moves and flattens the nucleus in an eccentric position. Since it is not surrounded by a membrane, the lipid drop represents a cellular inclusion. Functions of white adipose tissue include, besides energy storage, insulation, cushioning and protection of some structures, such as in the orbits and retroperitoneal space.
Brown adipose tissue
The cytoplasm of adipocytes in brown adipose tissue contains multiple lipid droplets (that is why it is described as multilocular). Its nucleus is central, round and the cytoplasm is clearly visible. The function of brown fat is to accumulate fat reserves which will later be depleted, mainly in thermal energy. Hibernating animals have large amounts of brown adipose tissue. In humans, it is abundant in newborns and protects them from low temperatures. The amount of brown adipose tissue gradually decreases as the body grows and can be found around kidneys, in interscapular, axillary and pubic regions.
Both types of tissue derive from a common progenitor cell, called preadipocyte, which is able to differentiate into white or brown fat.
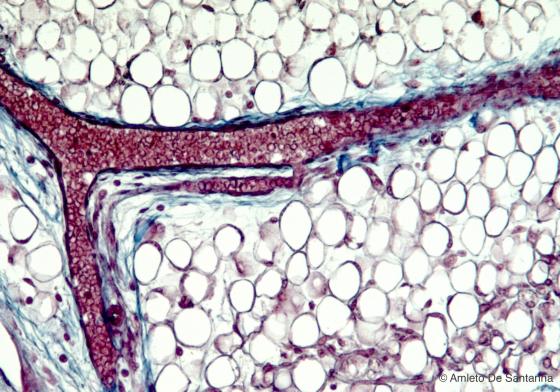
Figure C44. Human fetal mandible. White (unilocular) adipose tissue. The adipose cell is almost completely occupied by a large lipid droplet, the cytoplasm is a thin rim around the lipid droplet and the nucleus is flattened in the periphery. Since the lipid droplet has been solubilized during the sample preparation with alcohol and organic solvents, what remains in the adipocyte is a colorless area. Mallory-Azan X160
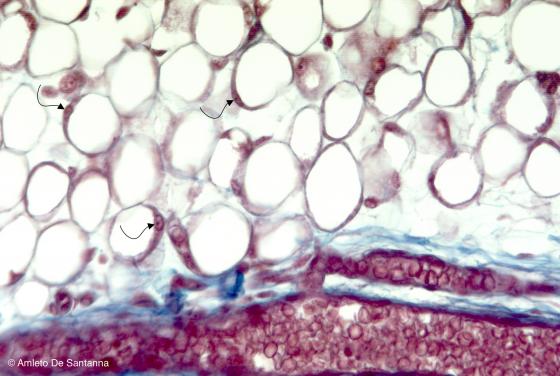
Figure C45. Human fetal mandible. White (unilocular) adipose tissue at higher magnification. You can see the nuclei of the adipocytes (arrows). Mallory-Azan X200
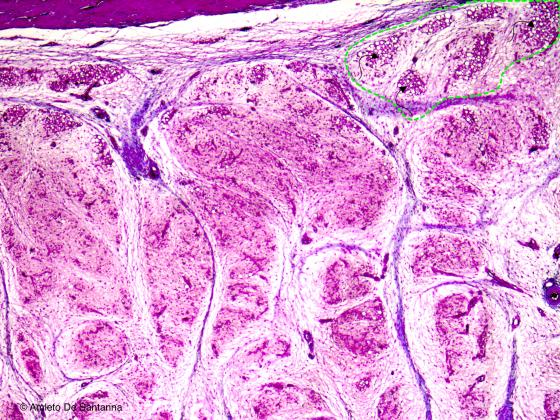
Figure C46. Human fetal foot. Since fetal life, the brown (multilocular) adipose tissue is mixed with the white (unilocular) adipose tissue (arrows inside the dotted area). In adult life, it is present only in some specific regions of the human body and it is prone to transform into white adipose tissue. After a long fasting or after a long period at low temperatures this type of adipose tissue may increase in volume and consistency. H&E X64
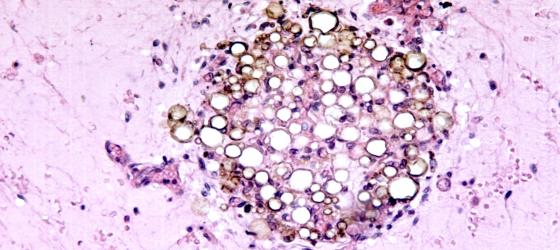
Figure C47. Adipose tissue in the mesentery of a marmot. You can see the white (unilocular) adipose tissue mixed with brown (multilocular) adipocytes. H&E X64
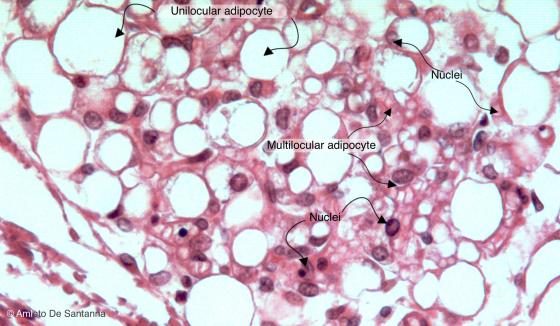
Figure C48. Adipose tissue of a marmot. You can see white (unilocular) adipose tissue, identifiable by the scant cytoplasm and the peripherally flattened nucleus of the adipocytes, along with adipocytes with a central roundish nucleus and an obvious cytoplams where there are several lipid droplets. These cells make the brown or multilocular adipose tissue. In mammals, this type of adipose tissue is normally present in the fetus, in the first months of life and in those animals that hibernate and must have a good reservoir of heat for a long period. In the other adult mammals, the brown adipose tissue is scarce. H&E X200
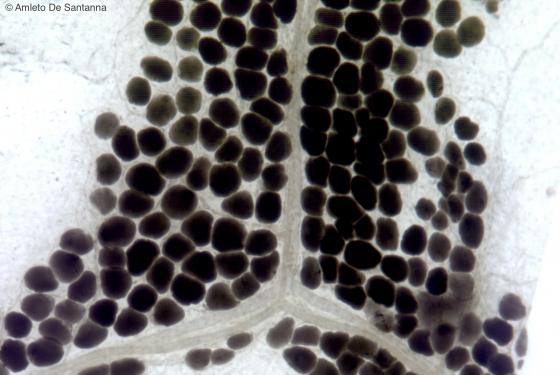
Figure C49. Human omentum. White (unilocular) adipose tissue that encloses a small blood vessel. This sample was frozen and cut with a cryostat at - 40°C, to avoid steps with solvents, like xylol or benzole, that would have solubilized the lipid droplet. Afterwars, the section was stained with the osmium tetroxide method. Osmium X64
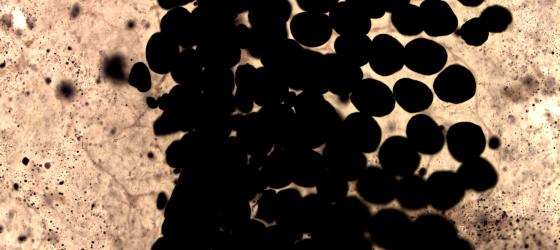
Figure C50. Human omentum. White (unilocular) adipose tissue that enclose a small blood vessel. This sample was frozen and cut with a cryostat at - 40°C, to avoid steps with solvents like xylol or benzole, that would have solubilized the lipid droplet. Afterwards, the section was stained with the Sudan Black method, a staining technique selective for the adipose tissue. Sudan Black X100
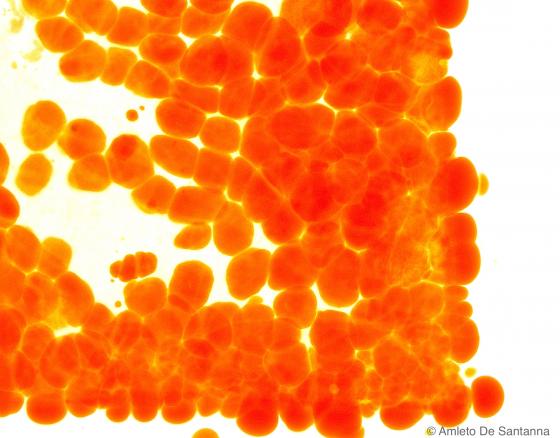
Figure C51. Mouse mesentery. Selective staining for adipose tissue in sections cut with a cryostat. Sudan IV X63
Cartilage
Cartilage is a specialized connective tissue, formed by chondroblasts and chondrocytes. Such cells are surrounded by a jelly extracellular matrix with fibers. Cartilage is the only connective tissue lacking in blood vessels: for this reason, it gets its nourishment through the permeability of the extracellular matrix.
Generally, cartilage is surrounded by the perichondrium, except in joints, where it is in direct contact with the synovial fluid. Chondroblasts are located in specific lacunae inside the extracellular matrix. where they can divide and create small cell groups known as isogenous groups, (surrounded by extracellular matrix.)
Depending on the extracellular matrix characteristics and functions, cartilage can be divided into:
- Hyaline cartilage
- Fibrocartilage
- Elastic cartilage
Hyaline cartilage
It undoubtedly is the most common cartilage in mammals. Its chondrocytes present a visible nucleus with one or more nucleoli. They are located in lacunae or depressions of the extracellular matrix. When hyaline cartilage is mature, chondrocytes tend to cluster in islets, the isogenous groups. They are more numerous in the deep zone of cartilage and tend to diminish in peripheral areas (intermediate zone and superficial zone). Isogenous groups are generally absent in immature hyaline cartilage. This cartilage is avascular and generally surrounded by the perichondrium. The matrix of hyaline cartilage lacks in fibers and is formed by an amorphous substance packed with proteoglycans, whose concentration is higher in the matrix surrounding isogenous groups (territorial matrix). The remaining matrix is characterized by fewer proteoglycans (interterritorial matrix).
Depending on the amount of acidic components (sialomucins, ialomucins), cartilage can be more or less basophilic. Therefore, from a staining point of view, it can be distinguished easily from other types of acidic connective tissues.
In addition to its supportive function, hyaline cartilage must provide flexibility to the skeleton.
Articular cartilage of long bone endings has no perichondrium. It has the shape of a lamina and presents a smooth surface, in order to facilitate the sliding of articular surfaces.
Metaphyseal cartilage (either growth or connective cartilage) can be found in long bones and is necessary for their length growth.
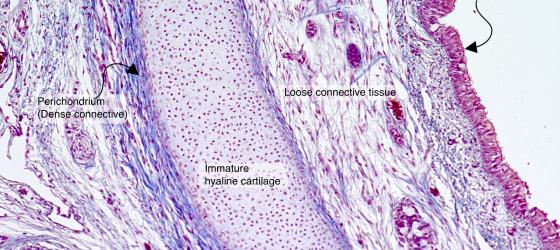
Figure C52. Human fetal trachea. Immature hyaline cartilage. Mallory-Azan X20
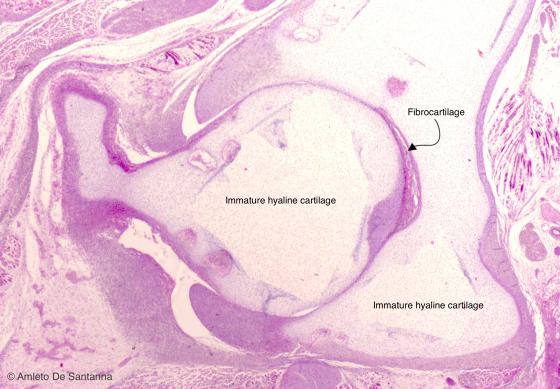
Figure C53. Human fetal coxofemoral joint. Immature hyaline cartilage. Transverse section through the coxofemoral joint. At low magnification, you can see the absence of isogenous groups, the morphology and the organization of the cells that make up the immature hyaline cartilage. H&E X25
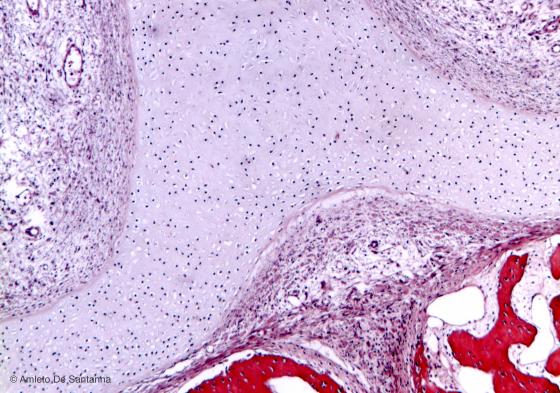
Figure C54. Nose of human embryo. Immature hyaline cartilage. Differently from mature hyaline cartilage, you can identify the immature hyaline cartilage because of the small dimensions of the lacunae, the absence of isogenous groups and, normally, because of the scarce staining of the extracellular matrix by conventional staining methods. H&E X40
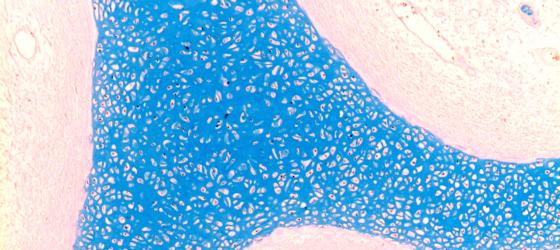
Figure C55. Nose of human embryo. Immature hyaline cartilage. Same sample of the micrograph shown in Figure C54 stained with a method selective for the acidic mucins that make up the majority of the extracellular matrix in the cartilage. Alcian blue X40
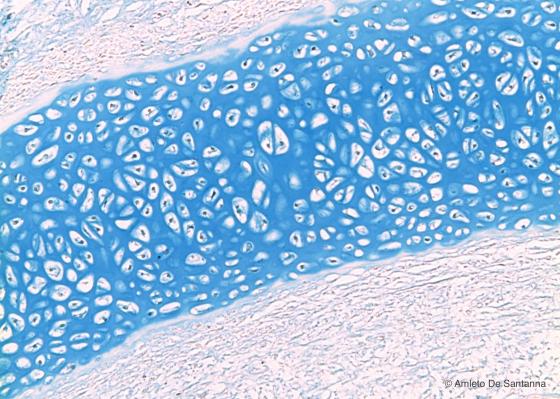
Figure C56. Nose of human embryo. Immature hyaline cartilage at higher magnification. At this magnification, you can see the low amount of extracellular matrix and the complete absence of isogenous groups and territorial matrix. Alcian blue X100
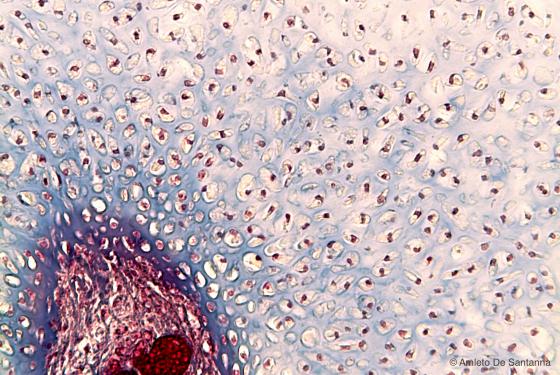
Figure C57. Foot of a human embryo. Degenerating immature hyaline cartilage with a secondary ossification center. Mallory-Azan X200
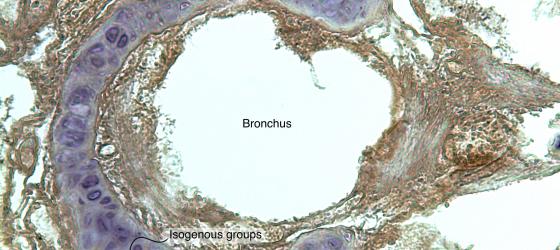
Figure C58. Dolphin lung. Pulmonary bronchus where you can see, stained purple, the hyaline cartilage that make up the rings and plates. DAB-E X100
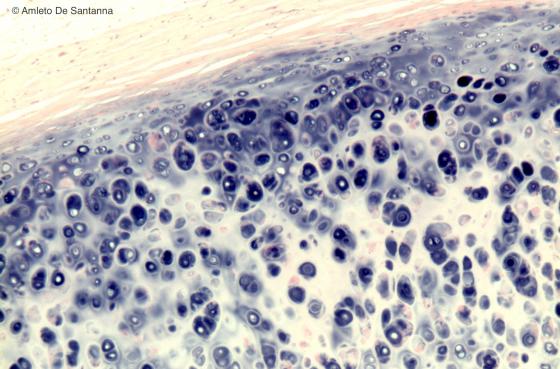
Figure C59. Human trachea. Hyaline cartilage. The architecture of the mature hyaline cartilage shows the isogenous groups, the darker territorial matrix and the lighter interterritorial matrix. Also, the perichondrium is obvious (stained light pink because it is strongly basic). On the contrary, the cartilage is the only connective tissue with an acidic extracellular matrix, stained blue-purple by hemalum (Mayer). H&E X63
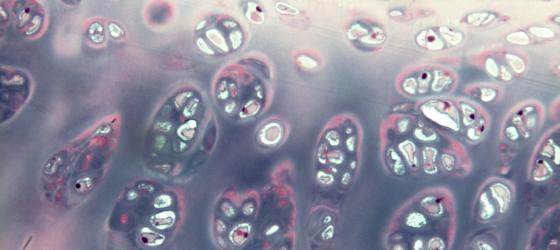
Figure C60. Human trachea. Mature hyaline cartilage. You can easily see the isogenous groups made up of several cells and the strong acidity of the cartilaginous matrix stained blue-purple by the hemalum. H&E X100
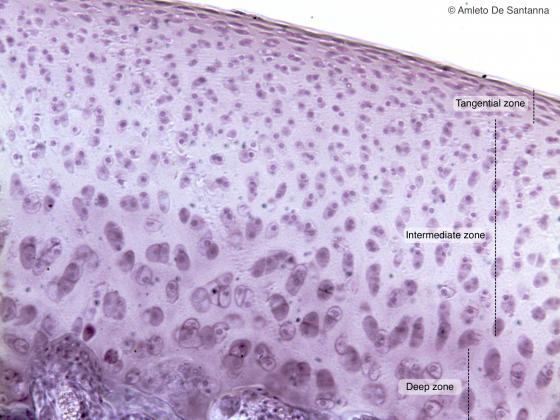
Figure C61. Human femur (head). Articular cartilage. You can see the growth of the cartilage by chondrocyte apposition. H&E X100
Fibrocartilage
It is formed by nearby plates of chondrocytes, lacking in ground substance and rich in fibers (type I collagen). It is generally avascular and does not present a perichondrium. Chondrocytes are isolated, in pairs, surrounded by extracellular matrix. Sometimes they are found overlapped in rows, between bundles of collagen fibers. This type of cartilage represents a transition from hyaline cartilage and dense connective tissue, so that there is no clear border between them. During embryonic development, as well as during various physiological conditions, it is possible that both tissues gradually turn into one another.
It can be found in some articular cartilages: intervertebral discs, articular discs of the menisci of the knee and mandibular joints, sternoclavicular joints and pubic symphysis. Moreover, it is present in the round ligament of femur and on bones, in the intersection areas with tendons.
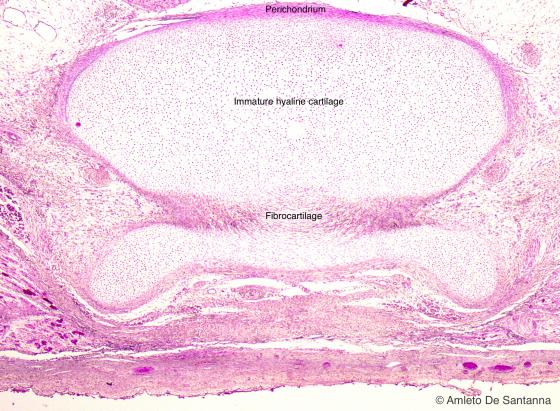
Figure C62. Human fetal vertebra. Longitudinal section of a vertebra where you can see the origin of the fibrocartilage. H&E X25
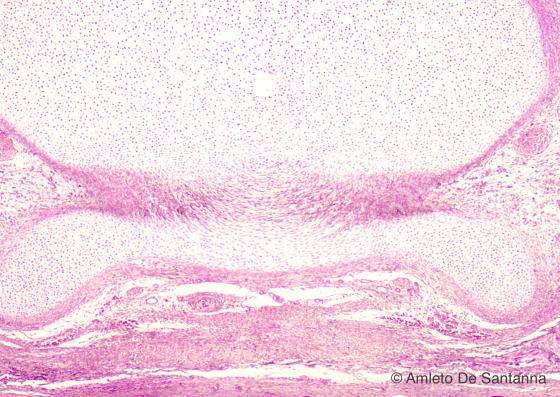
Figure C63. Human fetal vertebra. Longitudinal section of a vertebra at higher magnification. H&E X100
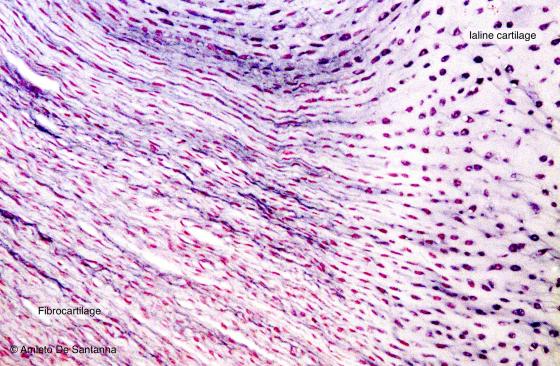
Figure C64. Human intervertebral disc. Transition zone from hyaline cartilage to fibrocartilage. The extracellular matrix of hyaline cartilage acquires a fibrous appearance and gradually loses its acidity and therefore the purple staining given by hemalum. In fibrocartilage, chondrocytes are smaller and less in number. Collagen fibers become longer and thicker, making up the fibrocartilage. H&E X100
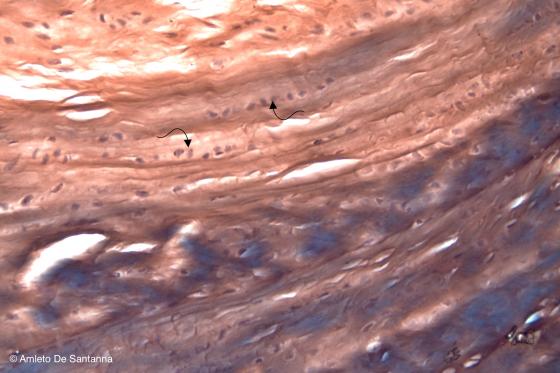
Figure C65. Human intervertebral disc. Chondrocytes are located inside lacunae that are aligned in parallel (arrows) and separated by dense collagen tissue. The most the tissue is fibrous the most the staining shift from purple, typical of the cartilage, to red-brown, typical of the collagen. H&E X100
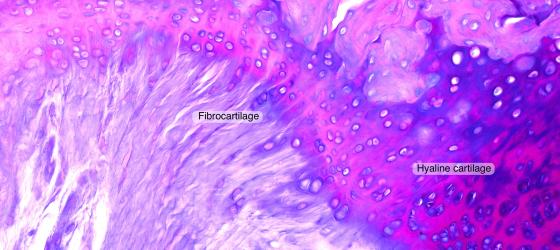
Figure C66A. Human fetal femur. Fibrocartilage. Alcian PAS X100
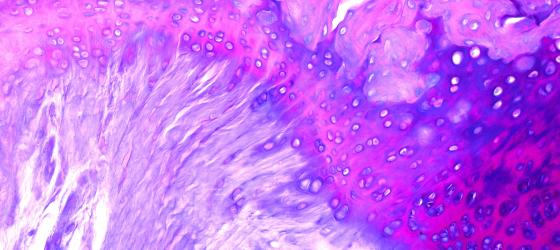
Figure C66B. Human fetal femur. Fibrocartilage. Alcian PAS X100
Elastic cartilage
The most prominent differences between elastic and hyaline cartilage refer to:
- The very little amount of extracellular substance, which lacks in amorphous component;
- The abundance of elastic fibers, which are interconnected especially in the deep zone, in order to create a network that surrounds the lacunae containing chondrocytes (this thin and branched elastic meshwork can easily be highlighted with Weigert’s resorcin-fuchsin).
- The lack of isogenous groups, formed by few cells.
Elastic cartilage is avascular and surrounded by perichondrium and it provides organs with higher elasticity (epiglottis, auricle, auditory or Eustachian tube).
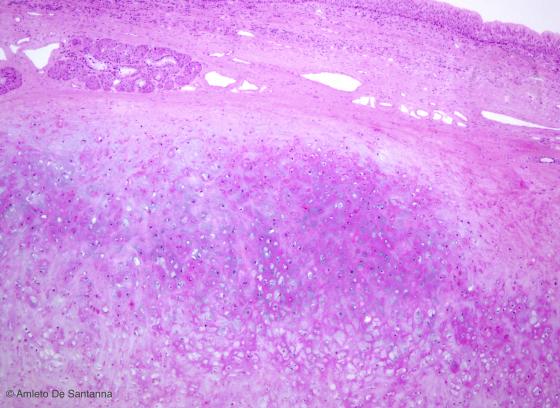
Figure C67. Human fetal femur. Fibrocartilage. With H&E staining, the elastic cartilage is recognized by the absence of isogenic groups even if the lacunae and chondrocytes are large. Another distinctive feature is the scarce presence of extracellular matrix in order to allow the elasticity of this tissue. Alcian PAS X100
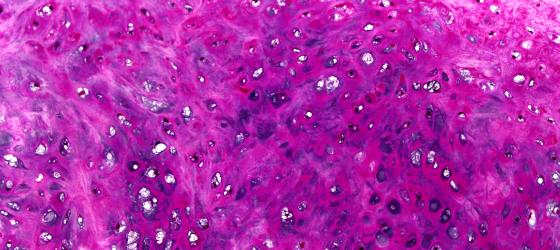
Figure C68. Human epiglottis. Digitally processed micrograph of elastic cartilage at high magnification. The lack of isogenous groups is obvious. X100
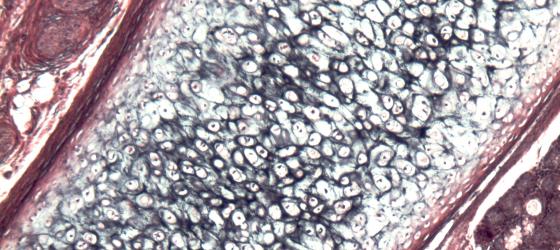
Figure C69. Human epiglottis. Elastic cartilage. Using a selective staining, you can see the cartilage elastic fibers stained black by Weigert’s resorcin-fuchsin staining. Weigert H&E X63
Cellular cartilage
It is composed of large, spherical, overlapping cartilage cells. There is a little amount of matrix which is rich in elastic fibers that oppose mechanical impulses, i.e. the auricular cartilage of rodents.
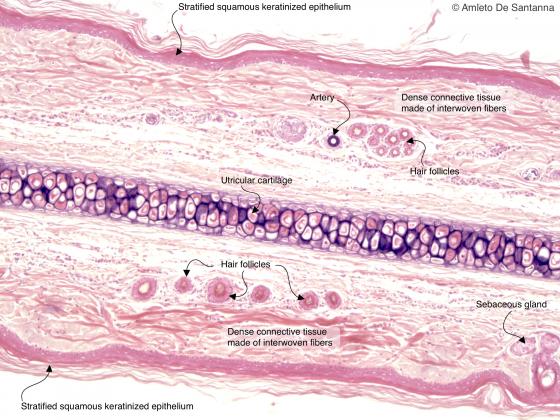
Figure C70. Mouse auricle. Weigert’s resorcin-fuchsin stains the elastic matrix of the cartilage black. This cartilage is called utricular cartilage because is found in mammal auricles. The resorcin-fuchsin stains also the tunica media of a small artery black. Weigert H&E X40
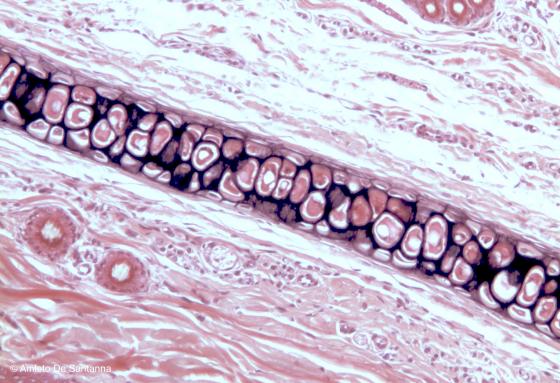
Figure C71. Rat auricle at high magnification. Elastic cartilage. Weigert H&E X100
Bone tissue
This tissue is dynamic and elastic. It can change its structure in response to organic and mechanical stimuli. It is formed by organic and inorganic components. The organic components are the typical cells of bone tissue (osteoprogenitor, osteoblasts, osteocytes and osteoclasts, responsible for bone development, growth, production and resorption, respectively) and the extracellular matrix (amorphous substance and type I collagen). As for the inorganic components, they are minerals such as calcium and magnesium phosphates and Na, Mn, K citrates that are located in the extracellular matrix. The organic component of extracellular matrix corresponds to 35% of the dry weight of the bone and it provides strength and elasticity, while the mineralized inorganic component corresponds to 65% of the dry weight and provides bone tissue with consistency and hardness. This tissue often changes its structure and function due to age, diet and the general conditions of the individual.
Cells of bone tissue
Osteoprogenitor cells
They derive from mesenchymal stem cells: they can proliferate and differentiate into osteoblasts. They are located in the periosteum and endosteum and once they are activated again, they are responsible for the development of new bone tissue.
Osteoblasts
Osteoblasts are osteocyte precursors. These cells are voluminous, highly polarized, their ovoid nuclei are slightly peripheral and have a highly basophilic cytoplasm. Osteoblasts guarantee organic matrix (osteoid) production as well as inorganic matrix deposition. Therefore, they have osteogenic functions and produce type I collagen, osteocalcin, osteopontin and bone sialoprotein.
Osteocytes
After bone development, when osteoblasts are trapped inside the lacunae within the matrix they produced, they become osteocytes. Their shape is irregular, their nuclei are elongated and clearly visible and their cytoplasm displays various extensions. They are located in bone lacunae from which multiple microscopic canaliculi branch off. Through these caniculi, osteocytes cytoplasmic extensions connect among themselves with communicating junctions and capillaries of bone canals. In this way, they allow metabolic exchanges among osteocytes and between osteocytes and blood. Osteocytes maintain the extracellular matrix of bone.
Osteoclasts
Osteoclasts are not related to the osteoprogenitor category, but they derive from the fusion of numerous monocyte progenitors (up to 30) and their function is to destroy (resorb) and remodel bone tissue. They are large cells and their diameter can exceed 100 µm. Moreover, they have multiple nuclei. Osteoclasts are highly polarized too: if they become activated, their cytoplasmic face near the bone is characterized by highly movable ripples. Osteoclasts adhere to the bone surface, giving rise to a microenvironment that is separated by the surrounding (sealing zone). This environment is acidified through the subsequent activation of enzymes, whose derivation can be both lysosomal and non-lysosomal (in the first case, proteinase and phosphatase, in the second case metalloproteinase). This process causes the erosion of bone matrix and develops a resorption bay known as Howship’s lacuna.
Types of bone tissue
There are two main types of bone tissue:
- Immature or nonlamellar bone, whose extracellular matrix does not present any lamellae; it is the primary bone tissue and it is present during the whole prenatal phase. In adults, it can be found in cases of recent bone deposition (for example, in case of fracture).
- Mature bone, that constitutes the majority of adult mammal bone tissue and is organized in lamellae.
Immature bone
During the prenatal phase and in some particular conditions in adults, the function of bone tissue is not to be resistant to pressure or stretch, but to be as light, elastic and plastic as possible.
This type of tissue presents a double organization: with interwoven or parallel fibers.
With interwoven fibers, collagen fibers are interwoven and form a dense meshwork. Their ground substance is disposed irregularly and in small amounts, both in the organic and inorganic part. Bone lacunae have a globular shape and are generally larger than in mature bone. Immature bone with interwoven fibers is present also in adult individuals, in sutures due to fractures, on the surfaces near the periosteum, in recent bone depositions in general and especially in cementum.
Conversely, immature bone with parallel fibers is rare in mammals: it can be found in tendon insertions.
Mature bone
Mature bone, due to its chemical composition and particular structure, is highly resistant to stretch, pressure and mechanical impulses in general. Indeed, due to its lamellar structure, this tissue offers a good resistance to impulses, without adding any weight to the skeleton.
Mature bone is divided into mature compact bone, when its composition is mainly formed by complete concentric lamellae, for example in diaphysis, and mature spongy bone. In this case, the structure is composed by incomplete lamellae forming several small fragments nestled among themselves (bone trabeculae), as for example in epiphyses.
Mature compact bone is very hard and contains numerous channels containing blood and lymph vessels which can be seen only with a microscope.
Mature spongy bone presents itself as a three-dimensional meshwork of bone trabeculae and delimits a maze-like space filled with bone marrow.
In long bones, epiphyses and diaphysis can be distinguished. The first ones are short, round, placed at the endings of the bone and constituted mainly by spongy bone. The second one is an elongated, cylindrical, central part and it is formed by compact bone; it is extremely resistant to impacts and pressure and contains bone marrow. In flat bones, on the other hand, there are two surfaces of compact bone tissue, called internal and external table. In the central area there is a layer of spongy bone tissue.
Mature compact bone
This tissue composes the diaphysis of long bones, the superficial layer of epiphyses, the tables of flat bones and covers all bone surfaces in general. Bone lamellae are organized in concentric layers that form osteons, in which osteocytes are placed circularly, according to different orbits, around the Haversian canals, containing one or two small capillaries. The number of lamellae surrounding a single Haversian canal varies from 4 to 20. The various Haversian canals communicate between them through other canals, placed transversally or obliquely, called Volkmann's canals, also containing capillaries. Nourishment reaches osteocytes through the capillary network and is distributed by the dense network of cytoplasmic extensions connecting them. The spaces between the various osteons are filled by mature bone fragments of variable shape and size, called interstitial systems. The borders among osteons and interstitial systems are easily detectable from a layer of refractive connective tissue, called cement line. The bone surface in contact with the periosteum and endosteum is formed by lamellae parallel to the free bone surface; these lamellae are called circumferential lamellae.
Mature spongy bone
It is formed by incomplete lamellae forming trabeculae (as for example in the epiphyses of long bones). This spatial disposition guarantees a better resistance to multidirectional pressures and creates intercommunicating spaces in which bone marrow is located. These lamellae can be more or less interwoven and have a variable thickness. Osteocytes are distributed irregularly in lamellae and have variable size and shape.
Periosteum
The external surfaces of bones are covered with a thick capsule of dense connective tissue with interwoven fibers, the periosteum. Its aim is to protect the bone and support the trophic action allowed by its several blood vessels. In the external part of the periosteum there are few cells and several collagen fibers (fibrous layer), while in the internal part, fibers are lacking, but there are abundant blood capillaries and osteoprogenitor cells with osteogenic potential (osteogenic layer). Some connective fibers called Sharpey's fibers depart transversally from the periosteum and head towards connective tissue. Their aim is to firmly anchor onto the bone, while penetrating the external circumferential lamellae. The periosteum is neither present in joints nor in muscle and ligament insertions.
Endosteum
A layer of squamous cells and connective fibers that cover all bone surfaces (trabeculae of spongy bone, medullary cavities of compact bone, Haversian and Volkmann’s canals) forms the endosteum. Endosteum cells have a high osteogenic potential.
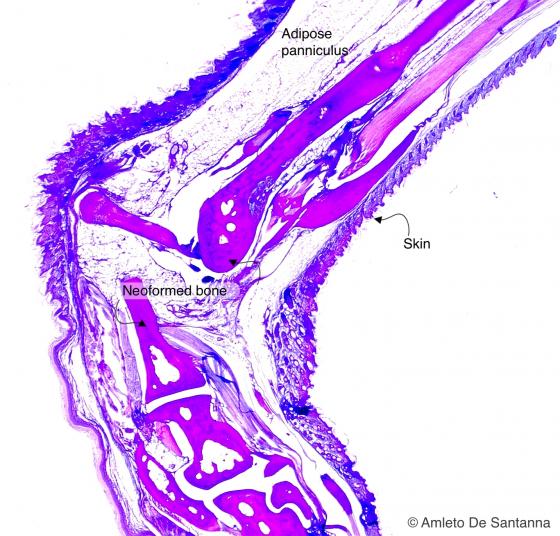
Figure C72. Mouse anterior limb. Alcian PAS X40
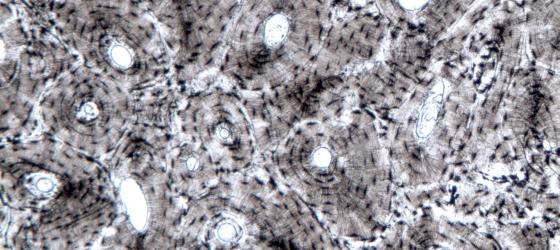
Figure C73. Human bone. Transverse section of unstained bone where you can see the lamellae of lamellar bone. X63
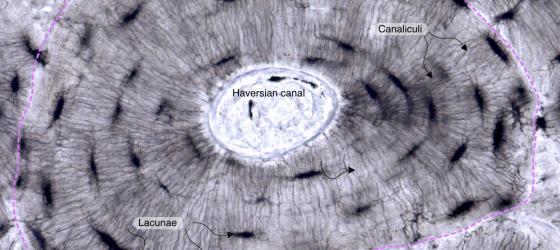
Figure C74. Human bone. Individual osteon at higher magnification (pink dotted line) where you can easily see the concentric lamellae that surrounds the osteonal (Haversian) canal. In the bone of a living organism osteocytes are interconnected by cytoplasmic processes. In this sample, you can see the pits where the osteocytes are located (lacunae) and the small tunnels (canaliculi) where the cytoplasmic processes run to connect the osteocytes. X160
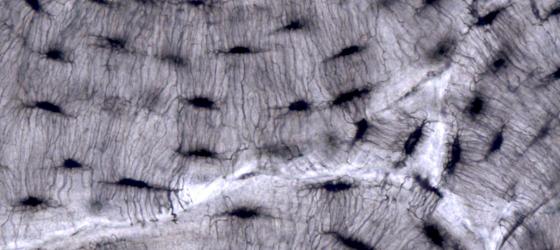
Figure C75. Human bone. Canaliculi at higher magnification. X400
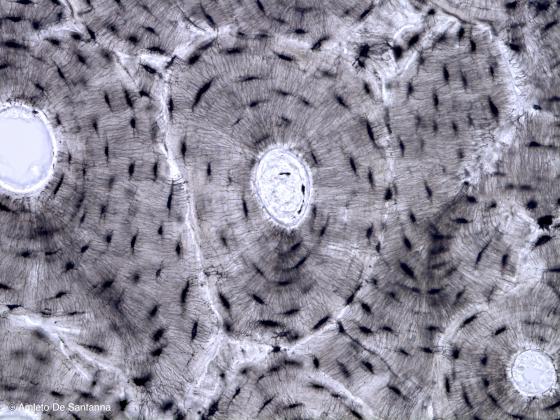
Figure C76. Human bone. Sample of lamellar bone obtained by rubbing and abrasive reduction of unstained bone. X100
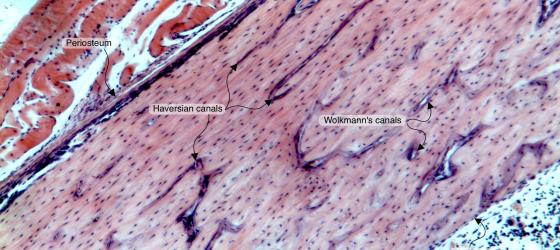
Figure C77A. Human femur. Lamellar bone in longitudinal section where you cannot see the osteons (or Haversian systems) but where you can clearly see both osteonal (Haversian) canals, longitudinally sectioned, and the perforating (Volkmann’s) canals in transverse section (since they run approximately at right angle to osteonal canals). H&E X40
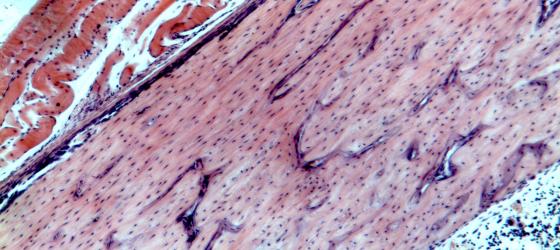
Figure C77B. Human femur. Lamellar bone in longitudinal section. H&E X40
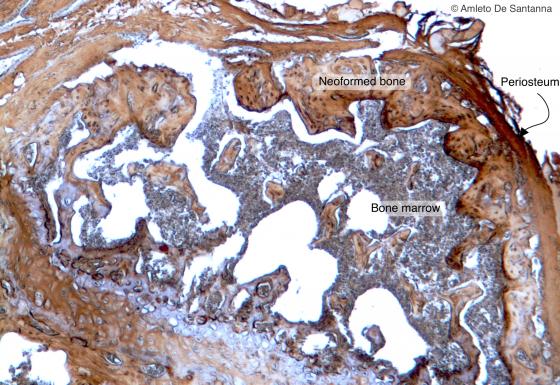
Figure C78. Mouse femur. Femur epiphysis cut transversely. Endochondral ossification has ended; you can see the trabeculae of the spongy bone with, inside, bone marrow. DAB-E X63
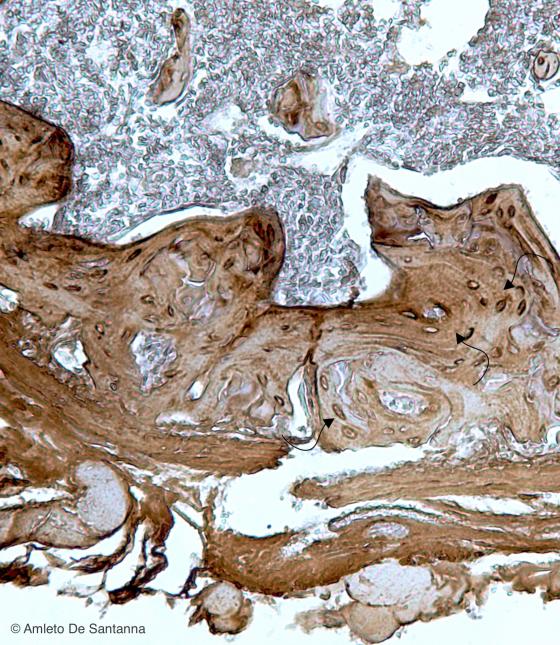
Figure C79. Mouse femur at higher magnification where you can see the lamellar organization of the trabeculae of the spongy bone (arrows). HRP-DAB X100
Osteogenesis
Osteogenesis, or ossification, is the process of new bone formation. It starts from preexisting mesenchymal tissue which is turned into bone tissue.
There are two types of osteogenesis:
- direct or intramembranous ossification, in which new bone tissue is created from mesenchymal tissue;
- indirect or endochondral ossification, in which new bone tissue is created from hyaline cartilage.
Both types of ossification create immature bone which is later converted into mature bone.
Direct ossification
During this process, the mesenchymal cells of the embryo aggregate in clusters (ossification centers) where they differentiate in osteoblasts deposing osteoid tissue (extracellular matrix of the bone), which is later mineralized in order to form immature bone with interwoven fibers. This tissue will subsequently be substituted by mature bone, after remodeling. Some examples of intramebranous ossification are frontal and parietal bones, as well as part of temporal and maxillary bones.
In the mandible, direct ossification process (mantellar ossification) begins with a cartilage bouton, a catalyst that enables the transformation of the surrounding mesenchymal tissue into bone tissue. In murine models, surgical removal of the cartilage bouton causes the lack of ossification of the mandible.
Indirect or endochondral ossification
When the ossification process takes place through an intermediate cartilage passage, it is called indirect or endochondral ossification. This is the typical ossification of spine, pelvis, part of the facial bones and ribs, and of long bones in general. The mechanism that gives rise to such ossification is called “substitution bone”. The calcified bone approximately presents the same structure of the preceding cartilage model, with significant variations only in size.
There are two processes that give rise to indirect ossification:
- In the cartilage model, the perichondrium on the diaphysis (the long part of the bone) thickens and in this way the number of osteoblasts present in the connective capsule increases. Subsequently, some blood vessels start penetrating the cartilage. They transport other osteoblasts and chondroclasts whose function is to destroy cartilage. A thin reticular meshwork generates from the external towards the internal part of the diaphysis, and it will provide the first cast of osteoid tissue (primary center of ossification). The majority of cartilage degenerates as newly formed bone tissue starts depositing. This process takes place through the formation of an ossification front in which cartilage cells place themselves in columns, disperse chromatin and undergo hypertrophy until they degenerate. At the same time, osteoblasts on the opposite front deposit osteoid tissue in intracellular spaces. Such tissue will lately be organized in lamellae and will form real bone tissue. In this way, the bone marrow elongates.
- When the ossification of most of the diaphysis is complete, blood vessels first penetrate into one epiphysis (the proximal), and lately into the other one: in this way, they create secondary ossification centers. During this second phase, the deposition of osteoid tissue starts from the inner part of the epiphysis and proceeds towards the outer part, so that it allows bone growth in width. Whereas in the diaphysis, the already-formed bone is remodeled by macrophages. In this way, it is possible for osteoblasts of the endosteum to deposit more bone tissue. This will cause a thickening of the diaphysis itself. At the end of indirect ossification, two layers of epiphyseal cartilage will be reabsorbed, the lower first and the upper second.
Stages of indirect ossification
This process takes place with subsequent stages. A typical example of it is the ossification of long bone metaphysis. Different areas may be identified:
- Zone of reserve cartilage, formed by reserve chondrocytes bordering with the epiphysis.
- Zone of proliferation, formed by chondrocytes in proliferative phase that are disposed in columns.
- Zone of maturation, formed by chondrocytes enlarging their size.
- Zone of hypertrophy, where chondrocytes reach their maximum size; the extracellular matrix is progressively calcified, diminishing its permeability to nourishing substances.
- Zone of resorption, where matrix calcification leads to chondrocytes resorption. The spaces left by empty lacunae are occupied by blood vessels and osteoprogenitor cells that later aggregate on the remaining calcified cartilage, where they deposit immature bone with interwoven fibers which will subsequently turn into mature bone.
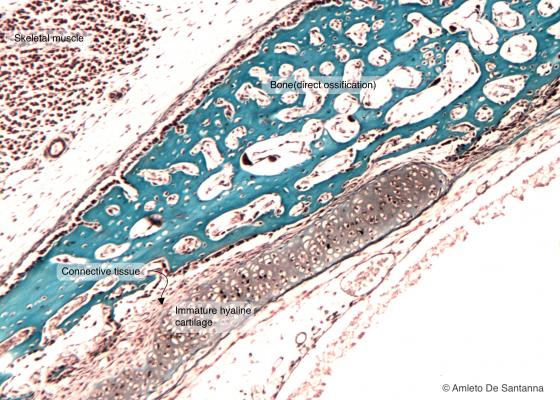
Figure C80. Human fetal skull. Intramembranous ossification of a flat bone. This type of bone formation consists in the direct transition from mesenchymal cells to bone (light blue). Mallory X63
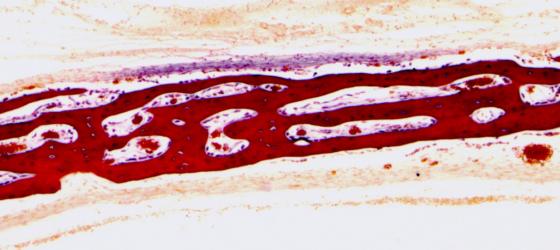
Figure C81. Human fetal skull. Intramembranous ossification of a flat bone at higher magnification. The bony trabeculae are stained red. Mallory-Azan X63
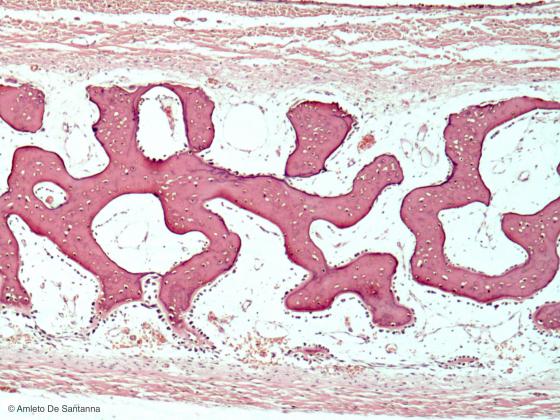
Figure C82. Human fetal skull. Intramembranous ossification of a flat bone. You can notice the complete lack of cartilage. H&E X63
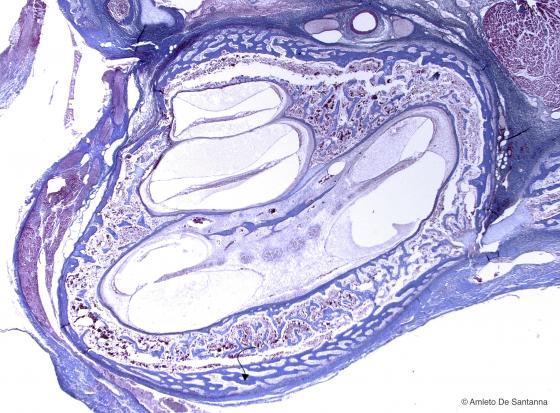
Figure C83. Human fetal temporal bone. Intramembranous ossification (arrow). Mallory-Azan X63
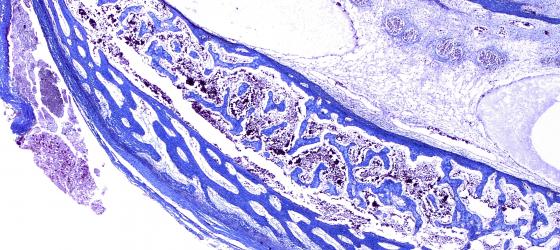
Figure C84. Human fetal temporal bone at higher magnification. Intramembranous ossification. You can see, stained blue, the osteoid tissue, newly formed directly from mesenchyme. Mallory-Azan X200
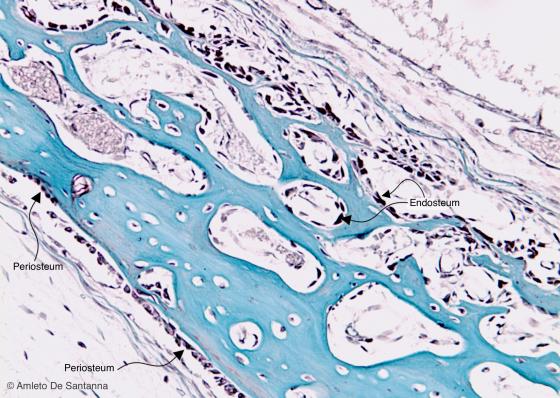
Figure C85. Human fetal skull. Intramembranous ossification. You can notice the external line (of the endosteum) and the external line (of the periosteum) made of osteoprogenitor cells. Mallory X100
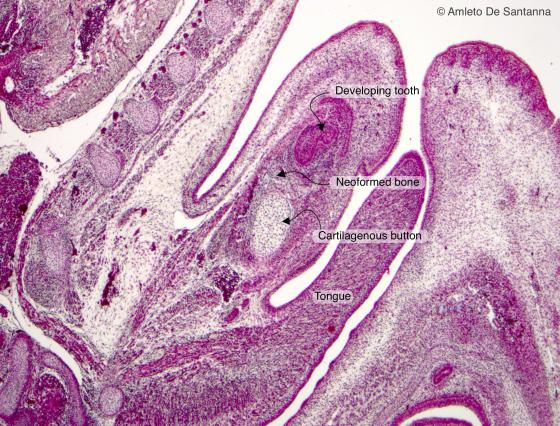
Figure C86. Mouse fetus. Direct mantellar ossification. This type of bone formation is specific of the mandible. This sample shows the beginning of the ossification process where you can see a well developed cartilaginous bouton and the mesenchyme starting to change into osteoid tissue. Mallory-Azan X25
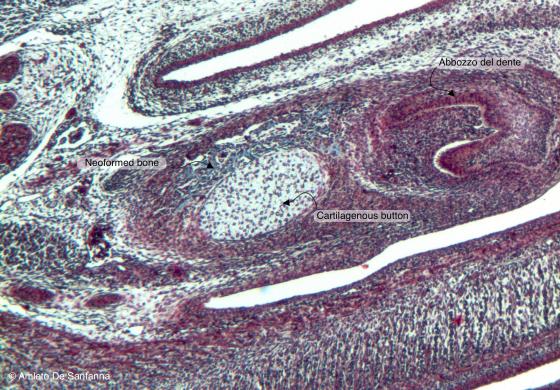
Figure C87. Mouse fetal mandible. Early phases of the mantellar ossification process. In the center, you can see the cartilaginous bouton, lighter, with on top newly formed bone tissue, in light blue. On the right, you can see a developing tooth. Mallory-Azan X63
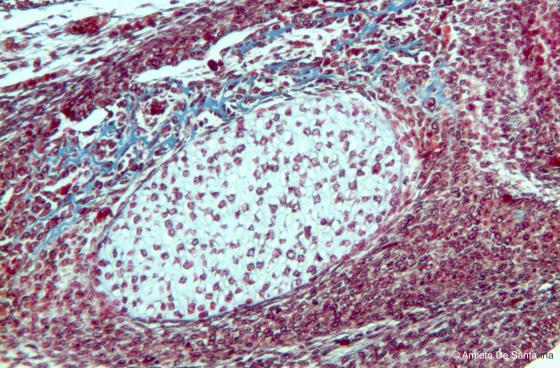
Figure C88. Mouse fetal mandible at higher magnification. You can see, stained light blue, the newly formed bone tissue that will form the mandible. Mallory-Azan X100
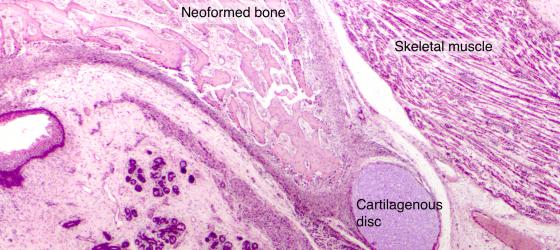
Figure C89. Human fetal mandible. Direct mantellar ossification in a later phase. H&E X25
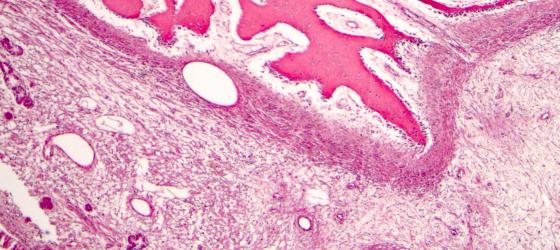
Figure C90. Human fetal mandible. Direct mantellar ossification in a fetus at term. You can see the cartilaginous bouton that is starting to decrease. H&E X40
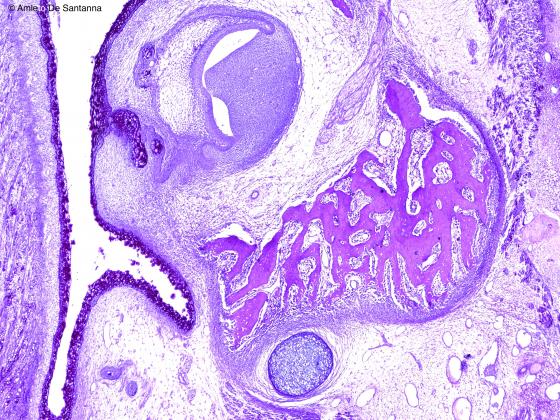
Figure C91. Human fetal mandible. Direct mantellar ossification stained with an immunohistochemical method to highlight the mucopolysaccharides of the cartilage (blue, acidic) and the bone (magenta). Alcian PAS X40
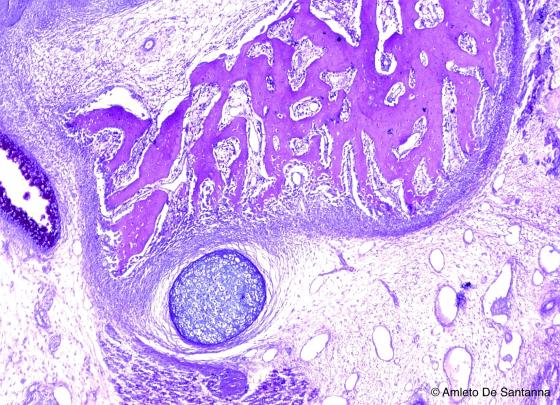
Figure C92. Human fetal mandible. Direct mantellar ossification at higher magnification, stained with an immunohistochemical method. Alcian PAS X63
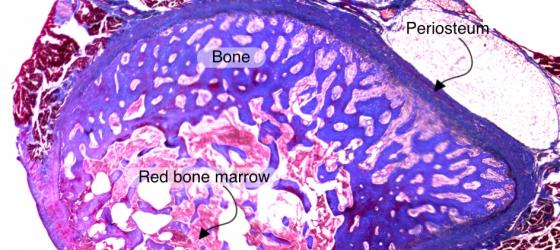
Figure C93. Human fetal rib. Direct (intramembraneous) ossification of a human rib. The rib ossifies both by direct (intramembraneous) and indirect (endochondral) ossification. To distinguish between the two types of ossification you need to see if there is continuity between cartilage and bone (indirect or endochondral ossification); or discontinuity between the tissues or lack of cartilage (direct or intramembraneous ossification). You can identify the osteoid tissue (not calcified immature bone tissue) because of the red staining of the azocarmine whereas the calcified bone is stained blue by the aniline blue. Mallory-Azan X40
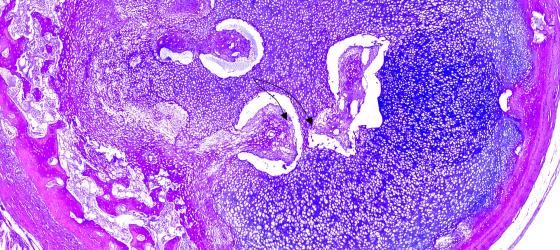
Figure C94. Human fetal rib. Indirect (endochondral) ossification of a human rib. You can see secondary endochondral ossification centers (arrows). Alcian PAS X25
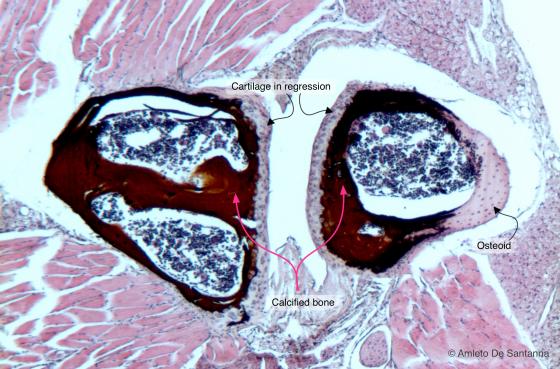
Figure C95. Mouse vertebrae. Not decalcified mouse vertebrae in the process of bone formation. Sample of vertebra with indirect (endochondral) ossification. You can notice the continuity between cartilage (in purple) and bone (in brown-black). The inorganic salts are highlighted by the brown-black staining of metallic silver precipitate. In the tissue regions stained pink, inorganic matrix is not formed yet. Von Kossa-H&E X63
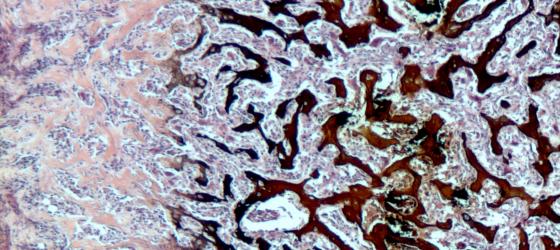
Figure C96. Human fetal tibia. Not decalcified transversely cut epiphysis of the tibia. On the right of the micrograph the spongy bone trabeculae are made up of mineralized matrix (stained brown-black), whereas on the left the trabeculae still lack inorganic material and therefore appear in light pink. Between the trabeculae there is red bone marrow. Staining method specific for the mineralized bone tissue. Von Kossa-H&E X63
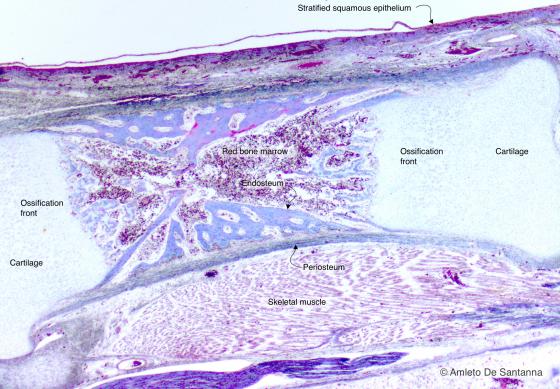
Figura C97A. Piede fetale umano. Ossificazione indiretta pericondrale delle ossa lunghe. L'ossificazione indiretta pericondrale è così denominata perché l'ossificazione procede a partire dal manicotto periostiale verso l'interno. Azan-Mallory 25x
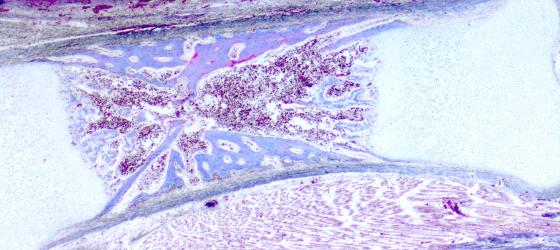
Figure C97B. Human fetal foot. Indirect ossification of long bones. The bone formation of long bones is defined indirect perichondral ossification because the bone formation starts from the periosteal bone proceeding inward. Mallory-Azan X25
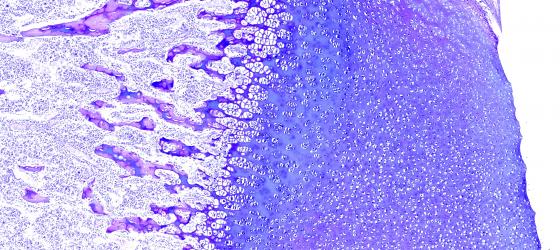
Figure C98. Human fetal foot. Indirect perichondral ossification of long bones. Alcian PAS X40
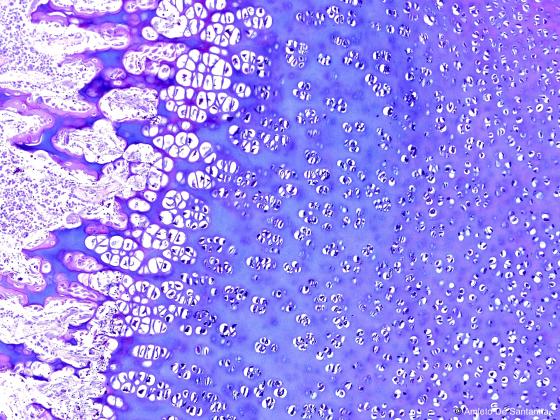
Figure C99. Human fetal foot. Indirect perichondral ossification of long bones at higher magnification. Alcian PAS X100
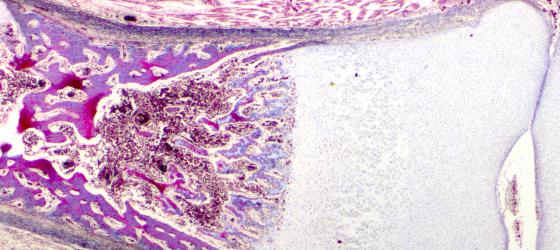
Figure C100. Human fetal foot. Indirect perichondral ossification of long bones. Mallory-Azan X40
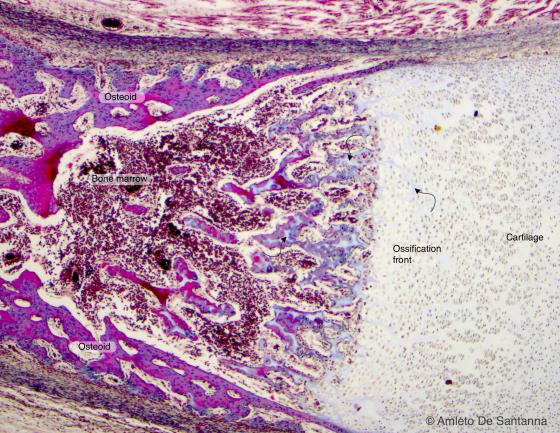
Figure C101. Human fetal foot. Indirect perichondral ossification of long bones at high magnification. The arrows point at the extracellular matrix decomposition. Mallory-Azan X63
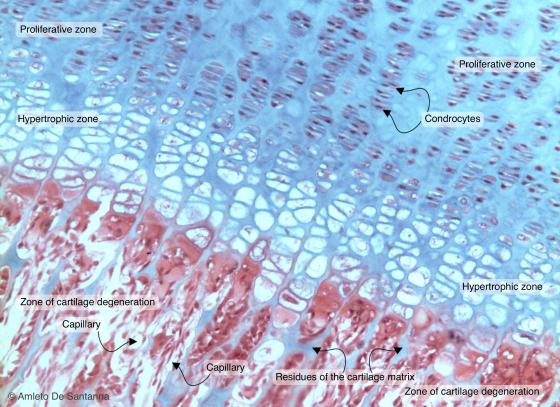
Figure C102A. Human femur. Indirect (endochondral) ossification. Ossification front. The micrograph shows: in the upper part, flattened chondrocytes piled to form columns (zone of proliferation); in the middle part, the ossification front made up of lacunae with necrotic chondrocytes and osteoblasts that secrete extracellular matrix (zone of hypertrophy); in the lower part, cartilage residues (in light blue) and the newly formed bone (in pink) surrounded by capillaries (zone of cartilage degeneration). H&E X100
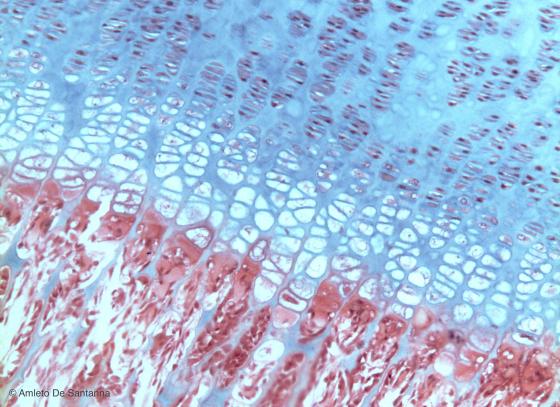
Figure C102B. Human femur. Indirect (endochondral) ossification. Ossification front. The micrograph shows: in the upper part, flattened chondrocytes piled to form columns (zone of proliferation); in the middle part, the ossification front made up of lacunae with necrotic chondrocytes and osteoblasts that secrete extracellular matrix (zone of hypertrophy); in the lower part, cartilage residues (in light blue) and the newly formed bone (in pink) surrounded by capillaries (zone of cartilage degeneration). H&E X10
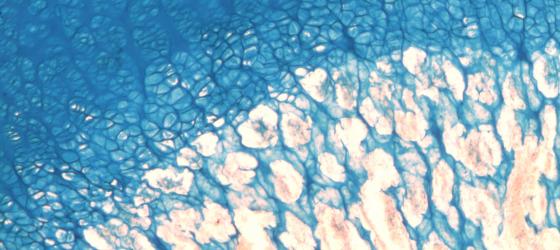
Figure C103. Human femur. Tangential section of the ossification front. In the upper part of the micrograph you can see the chondrocytes still organized in isogeneous groups and a strongly acidic extracellular matrix; in the middle part, the chondrocytes piled in columns and a weakly acidic extracellular matrix; in the lower part, the residues of acidic extracellular matrix of the cartilage (stained light blue) and the earliest formation of osteoid tissue (stained light pink). Alcian Blue X63
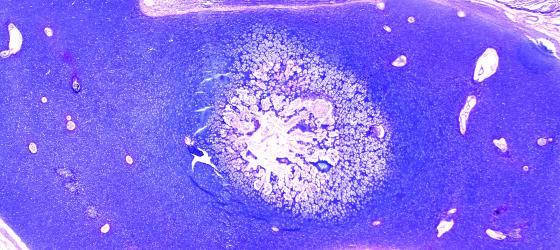
Figure C104. Human fetal heel. Indirect (endochondral) ossification. Alcian PAS X25
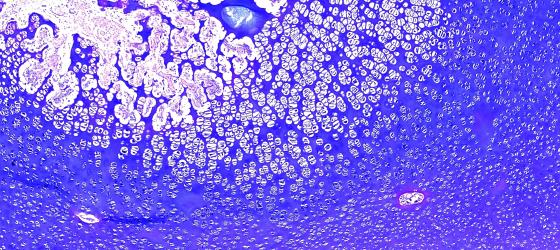
Figure C105. Human fetal heel. Indirect (endochondral) ossification. Alcian PAS X40
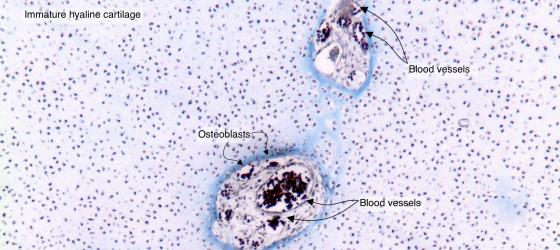
Figure C106. Epiphysis of a human long bone. Secondary ossification centers. Cartilage is the only connective tissue that is not vascularized, therefore if blood vessels are present there is ongoing bone formation. This section was obtained cutting transversely the inferior epiphysis of a tibia. In the center, you can see, dived in mature hyaline cartilage, the first centers of secondary endochondral ossification. Mallory-Azan X63
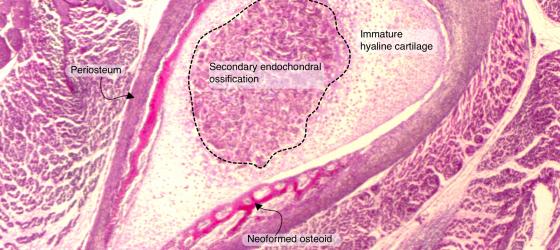
Figure C107. Human fetal femur (epiphysis). Indirect (endochondral) ossification. In long bones, the secondary endochondral ossification starts from the central part of the epiphysis widening progressively toward the periphery, allowing the bone to grow in width. H&E X25
Lymphatic tissue
This is a particular connective tissue, characterized by its structure: it is composed mainly by lymphocytes, cells supported by a dense connective network. The cells composing this tissue originate in organs called primary lymphatic organs. They move in the different parts of the body through blood and lymph circulation (therefore, in blood and lymph connective tissues); they can stop in other lymphatic organs, called secondary lymphatic. Finally, they can leave the vessels and migrate through loose connective tissue. What has been said helps to understand that the cells constituting lymphoid tissue can also be found in various types of connective tissue. This happens because their function is not the typical function of connective tissue (i.e. trophic and supportive), but a protecting function against various pathogens. This function relies on their ability to circulate throughout the body and reach the area containing a pathogen to destroy. They can be found either isolated or, more often, aggregated in more or less organized groups.
Lymphocytes are in general small cells, with a central, round, highly visible nucleus, surrounded by a thin cytoplasmic ring. Their main morphological characteristic, other than a small size, is their high stainability, regardless of the technique.
Lymphatic organs are divided into primary lymphatic (or central) and secondary lymphatic (or peripheral) organs.
In humans, primary lymphatic organs are the bone marrow and the thymus (in fetuses, the liver and the spleen). Birds have a particular primary lymphatic organ, located in the intestine, called the bursa of Fabricius. Secondary lymphatic organs are the spleen, lymph nodes, and the mucosa-associated lymphatic tissue (MALT: tonsils, Peyer’s patches, cecal appendix, and other lymphocyte clusters of the mucosa).
All the differentiation stages take place in the primary lymphatic organs. Such stages start from already lymphatic-oriented stem cells and produce mature virgin lymphocytes (B lymphocytes in the bone marrow; T lymphocytes in the thymus) (antigen-independent process). After lymphocytes meet the antigen (antigen-dependent activation), they start complying with their functions in the secondary lymphatic organs.
There are two main types of lymphocytes:
- B lymphocytes, are generated and matured in the bone marrow (in birds they generate and mature in the bursa of Fabricius, hence their name);
- T lymphocytes are generated in the bone marrow, but reach their maturation in the thymus (hence their name).
There is a third category of lymphocytes. Initially, they were defined as neither B nor T cells because they do not have the same phenotypic features. They are known as Natural Killer lymphocytes (NK).
Once the maturation process is over, lymphocytes migrate towards the secondary or peripheral lymphatic organs, such as the spleen, lymph nodes and the mucosa-associated lymphatic tissue (MALT) in order to comply with their functions and recognize and fight microbial infections. The spleen not only carries out an immune function, but also destroys immature or malfunctioning red blood cells (hemocatheresis). This process takes place in the red pulp.
Bone marrow
In humans, this is the main hemopoietic organ. The bone marrow is formed by lymphatic tissue and it is named after its location: the inner cavities of both long and flat bones. In normal conditions, it has a primary function in the production and maturation of blood cells. The bone marrow with hematopoietic function is called red bone marrow because, in vivo, it appears with an intense red color, due to the high presence of blood and erythrocytes. During the first moments of the fetus life, it first appears in the clavicle and subsequently spreads to all the remaining bones. During fetal development and for several years, the red bone marrow is prevalent. After the individual develops, the majority of bone marrow loses its hemopoietic function, while the adipocyte and connective component increases. This involves a change in color, which turns white-yellowish. The result is yellow bone marrow. In adults, this change narrows the hemopoietic function, and hence the presence of red bone marrow, to some zones of the cranial diploë, and to sternum, ribs, iliac crests and the central areas of some short bones.
Bone marrow includes:
- the supportive tissue, formed by connective cells, reticulocytes, that form a dense reticular meshwork with their argyrophilic fibers. Such meshwork supports the cells of the hemopoietic tissue and forms a spongy reticular system that eases their passage and migration;
- the hemopoietic compartment, packed with differently matured cells (erythrocytes, neutrophil granules, basophilic granules, eosinophilic granules, lymphocytes, monocytes, megakaryocytes, platelets);
- the vascular compartment, constituted by venous sinuses or sinusoids that allow a constant migration of erythrocytes and other elements through the circulation as well as having a trophic function. Leaning against the sinusoids are the megakaryocytes, large cells that through the budding and detachment of a part of cytoplasm create platelets.
It is difficult to obtain bone marrow histological preparations due to the extremely delicate structure of this tissue. After an adequate fixation and decalcification of the bone tissue, microtome sections can be achieved, as well as compression preparations or preparations with tissue fragment smears. The most used techniques are those typically used for blood staining: Em-Eo o May Grumwald-Giemsa.
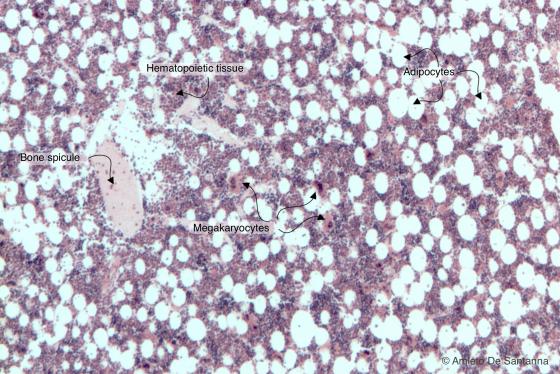
Figure C108A. Human red bone marrow. The hematopoietic tissue is stained red-purple; adipocytes are colorless since the lipid droplet has been solubilized by solvents. H&E X40
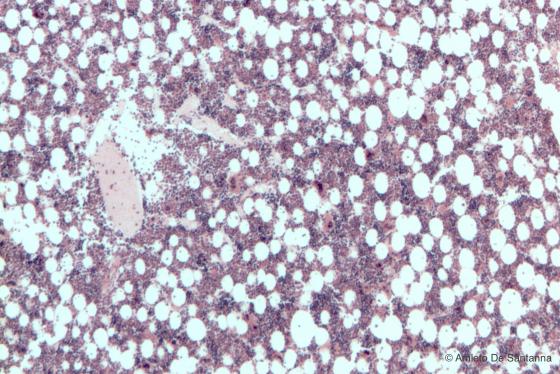
Figure C108B. Human red bone marrow. The hematopoietic tissue is stained red-purple; adipocytes are colorless since the lipid droplet has been solubilized by solvents. H&E X40
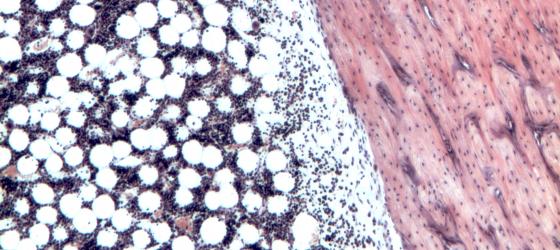
Figure C109. Human bone marrow in situ. Longitudinal section of a long bone with inside red bone marrow composed of hematopoietic tissue mixed with adipose tissue. H&E X63
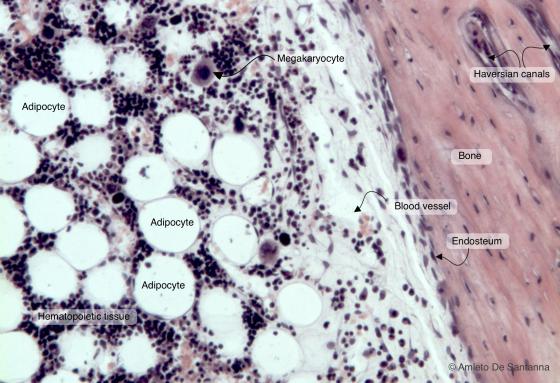
Figure C110A. Human bone marrow in situ at higher magnification. You can clearly see both the endosteum that lines the lamellar bone and some large megakaryocytes inside the hematopoietic tissue. H&E X100
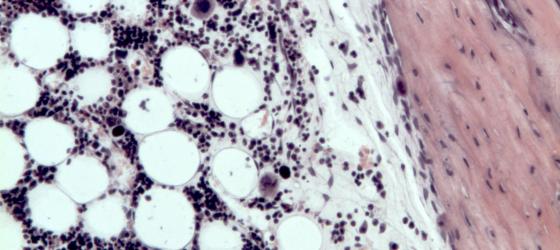
Figure C110B. Human bone marrow in situ at higher magnification. You can clearly see both the endosteum that lines the lamellar bone and some large megakaryocytes inside the hematopoietic tissue. H&E X100
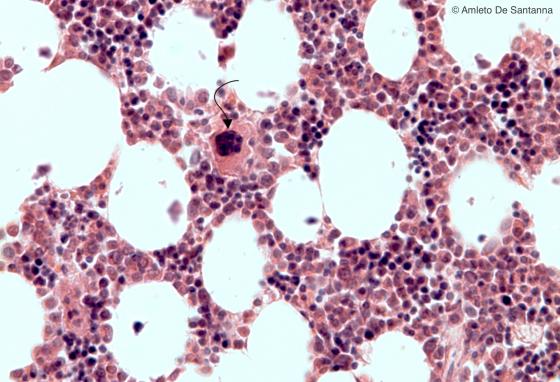
Figure C111. Human bone marrow at high magnification. You can see islets of hematopoietic tissue mixed with adipocytes. In the center of the micrograph, you can see a large cell with a polylobed nucleus: the megakaryocyte (arrow), from which platelets originate by budding cytoplasm portions. H&E X200
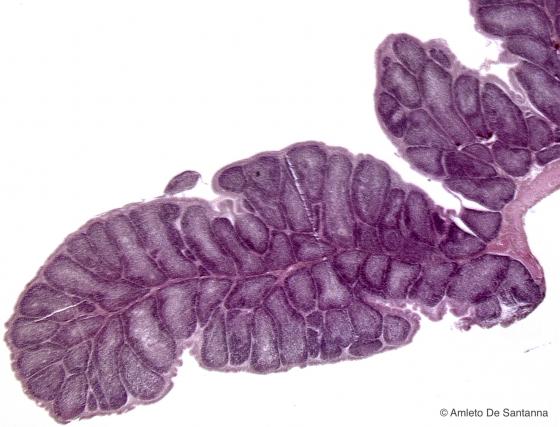
Figure C112. Bursa of Fabricius. The bursa of Fabricius is a primary lymphoid organ present only in birds and located in the wall of the intestine. Iron hematoxylin-eosin X12.5
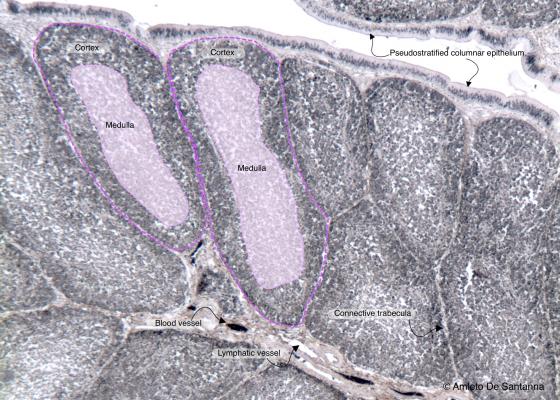
Figure C113A. Bursa of Fabricius. Nonetheless, the bursa of Fabricius has a morphological appearance similar to the thymus; it produces B lymphocytes (the B of B lymphocyte derives from Bursa). Iron hematoxylin-eosin X63
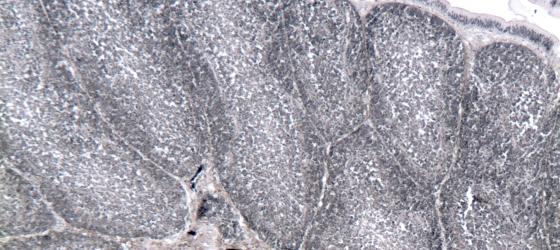
Figure C113B. Bursa of Fabricius. Nonetheless, the bursa of Fabricius has a morphological appearance similar to the thymus, it produces B lymphocytes (the B of B lymphocyte derives from Bursa). Iron hematoxylin-eosin X63
Thymus
This organ is located in front of the heart large vessels. It is formed by two lobes and morphologically characterized by a thick connective capsule, from which various septa leave and divide the organ into lobules. Each lobule contains a peripheral zone, called cortical, with a more intense color, in which thymocytes are more numerous and separate, and a lighter zone, called medullary, centrally located, where thymocytes are less numerous and where some formations called Hassall’s corpuscles are present. Hassall’s or thymic corpuscles are round formations of around 30-100 µm composed of medullary epithelial cells, keratinized and concentrically arranged. This is the reason why they appear highly colored with basophilic staining methods (for example, in HE with eosin intense pink).
The thymus is extremely active during childhood: starting from the teenage years, it regresses and leads to a morphological substitution, with a more gradually confused organization, rich in connective and adipose tissue. In adults, the thymus is represented by a few portions of lymphatic tissue surrounded by connective tissue and adipocytes.ti.
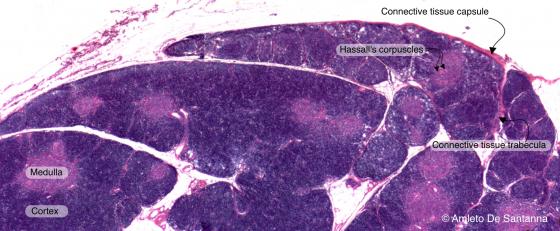
Figure C114A. Human thymus. The thymus is a primary lymphoid organ filled up with cells called thymocytes that subsequently mature into T lymphocytes. The connective tissue capsule sends inward septa that divide the organ into lobules, each one containing an external darker cortex and an inner, less stained, medulla. H&E X10
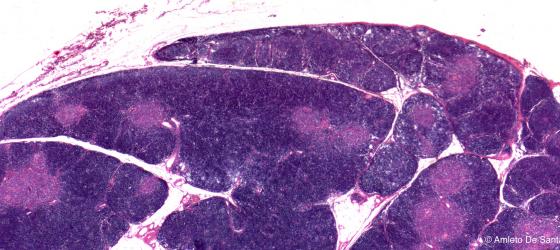
Figure C114B. Human thymus. The thymus is a primary lymphoid organ filled up with cells called thymocytes that subsequently mature into T lymphocytes. The connective tissue capsule sends inwards septa that divide the organ into lobules, each one containing an external darker cortex and an inner, less stained, medulla. H&E X10
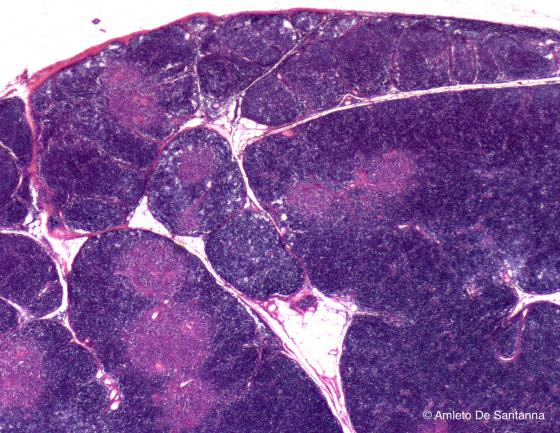
Figure C115. Human thymus at higher magnification. The connective tissue capsule sends inwards septa that divide the organ into lobules, each one containing an external darker cortex and an inner lighter medulla. H&E X40
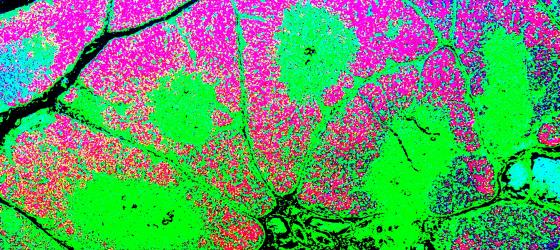
Figure C116. Human thymus at higher magnification. Digitally processed micrograph. You can see the medulla in light green, the connective septa in dark green and the cortex in deep pink. X40
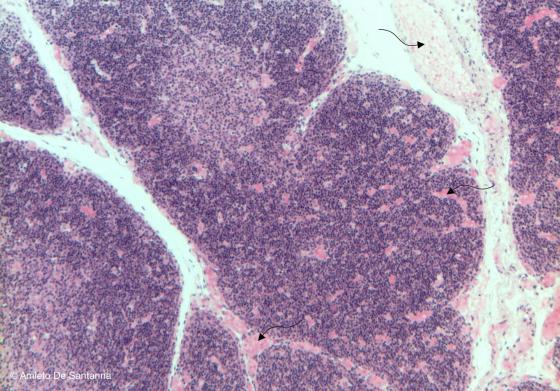
Figure C117. Human thymus. The thymus is easily identifiable as a lymphoid organ because of the subdivision into lobules, the large amount of lymphocytes organized into a cortex and a medulla and the concentric eosinophilic formations in the center of the medulla and named thymic corpuscles. You can notice the large amount of blood vessels stained pink (arrows). H&E X63
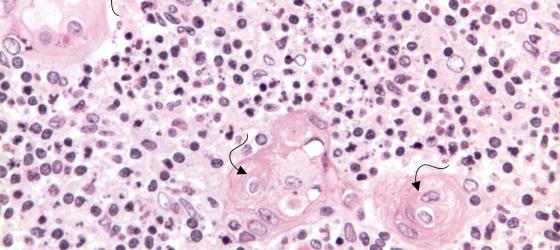
Figure C118. Human thymus. In the medulla, the epithelioreticular cells may have a concentric organization that lead to the formation of corpuscles called thymic corpuscles or Hassal’s corpuscles (arrows). These cells show clear signs of degeneration. H&E X200
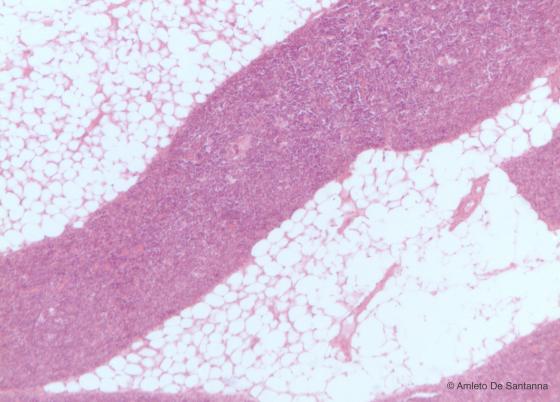
Figure C119. Thymus in regression of an adult subject. The thymus morphology, after puberty, progressively change: the lymphoid tissue decreases while adipocytes and connective tissue increase. E&H X40
Spleen
The spleen is a large lymphatic organ located in the abdominal cavity on the left, under the diaphragm. It is surrounded by a thick connective capsule from which several and thick septa leave. Although they penetrate deeply into the organ, they do not split it into lobules. Rather, they become thinner and thinner until they form a thin reticular meshwork that supports the parenchyma. The spleen has various main functions: it organizes the immune response against blood microbes (lymphopoietic function, white pulp) and destroys malfunctioning or deteriorated erythrocytes (hemocatheretic function, red pulp).
The parenchyma of the spleen is divided into red pulp and white pulp with the interposed marginal zone, which presents several arteries and active macrophages.
White pulp is characterized by lymphatic nodules, ovoid formations composed of B lymphocytes, and periarterioral sheaths formed by T lymphocytes. The arteriole is generally central with respect to the periarterioral sheath. In histological specimens, the white pulp is easily distinguishable due to its darker shade, both with HE and with other conventional staining methods, because of the high affinity of lymphocytes with acidophilic staining methods.
Red pulp is composed of a dense network of sinusoids surrounded by endothelial cells, macrophages and plasma cells, and it is uniformly distributed inside the organ. Under the microscope, this area is easily recognized because of its light color, lower concentration of lymphocytes and higher quantity of loose connective tissue.
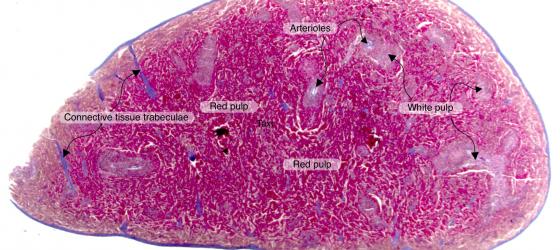
Figure C120A. Mouse spleen at low magnification. The spleen is a secondary lymphoid organ. The spleen parenchyma presents a white pulp and a red pulp. The white pulp derives its name from the fact that the lymphoid tissue of the periarteriolar sheets is white whereas the red pulp, that is highly vascularized, is dark red. Mallory-Azan X12.5
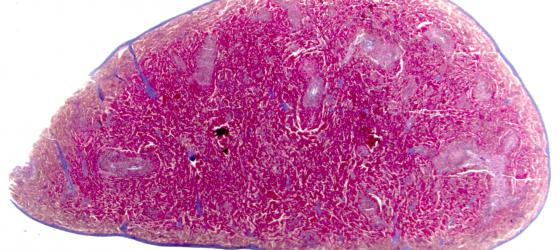
Figure C120B. Mouse spleen at low magnification. The spleen is a secondary lymphoid organ. The spleen parenchyma presents a white pulp and a red pulp. The white pulp derives its name from the fact that the lymphoid tissue of the periarteriolar sheets is white whereas the red pulp, that is highly vascularized, is dark red. Mallory-Azan X12.5
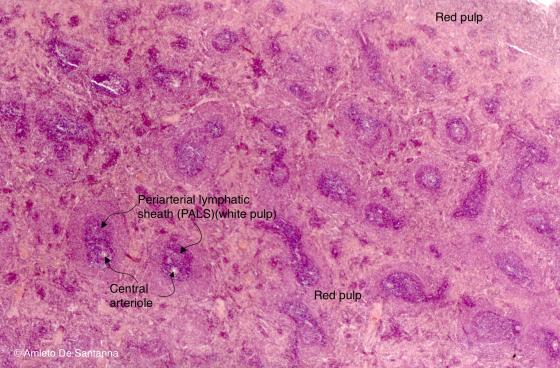
Figure C121A. Mouse spleen. In the spleen, a secondary lymphoid organ, is evident the division of the parenchyma in a white pulp, made up of periarteriolar sheets, and a red pulp. H&E X25
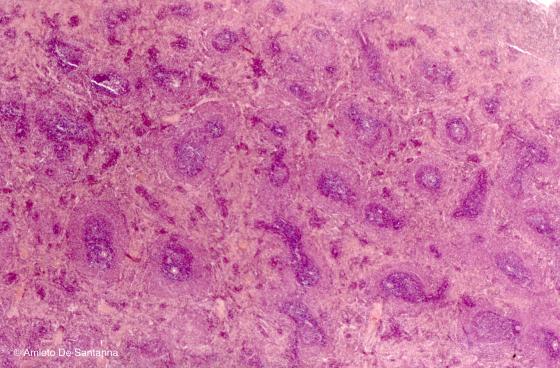
Figure C121B. Mouse spleen. In the spleen, a secondary lymphoid organ, is evident the division of the parenchyma in a white pulp, made up of periarteriolar sheets, and a red pulp. H&E X25
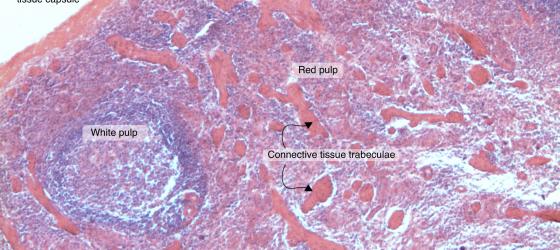
Figure C122. Mouse spleen. You can see, stained pink-orange, the connective tissue capsule that projects inwards large connective septa, without dividing the organ into lobules. H&E X63
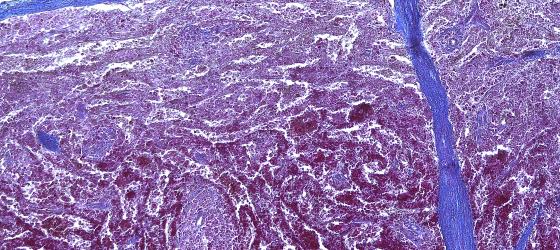
Figure C123. Human spleen. Azan three-color process shows the connective tissue capsule, stained light blue, that encloses the organ and the septa that project inwards to support, together with the reticular connective tissue, the parenchyma. Mallory-Azan X40
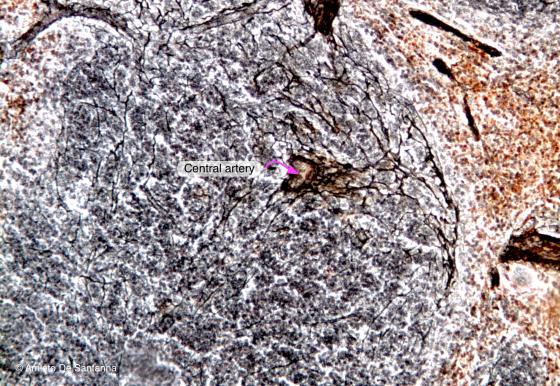
Figure C124A. Human spleen. Reticular fibers that enclose a lymphoid follicle and the central artery. Bielschosky X100
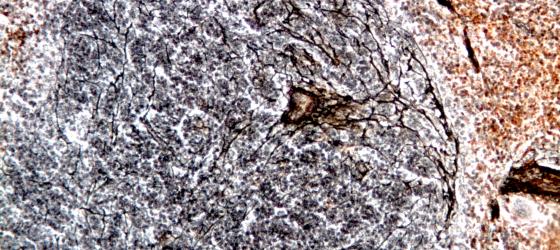
Figure C124B. Human spleen. Reticular fibers that enclose a lymphoid follicle and the central artery. Bielschosky X100
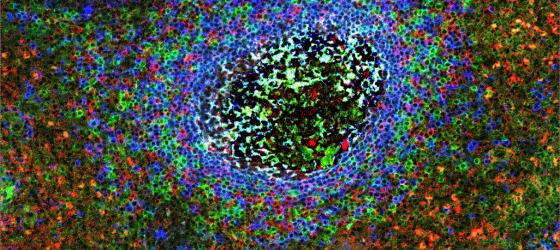
Figure C125. Human spleen. Secondary lymphoid follicle. The B lymphocytes making up the follicle have been stained with anti-IgG (red), anti-IgM (blue) and anti-IgA (green) antibodies. You can see also some small blood vessels. (Courtesy of Silvia Bruno, Department of Experimental Medicine, University of Genoa).
Lymph nodes
In normal conditions, lymph nodes are small, ovoid organs distributed along the lymph vessels. The lymph node is surrounded by capsular dense connective tissue that sends septa that later penetrate the lymph node parenchyma for a short distance. Although these septa are clearly visible, they rarely manage to reach the medulla. The parenchyma is composed of lymph cell accumulations supported by a stroma composed of a dense network of reticular connective tissue and divided into three zones: the cortex, packed with B lymphocytes, the paracortex, packed with T lymphocytes, and the medulla, packed with plasma cells.
The cortex is the most superficial zone. It is located underneath the connective capsule and is composed of numerous ovoid formations called follicles. When follicles present a constant morphology and a uniform color are called inactive primary follicles, while when they present a clearer central area (germinal center of Fleming) surrounded by a darker area (the mantle), they are known as active secondary follicles.
Deeper to the follicle zone is the paracortex, rich in T lymphocytes that are squeezed in order to create a dense network in which follicles are rare.
In the center of the lymph node there is the medulla, less colored than the cortex because of the high amount of vessels and connective tissue. Here, lymphocytes are clustered around the medullary cords. They are branched and tend to interconnect. Inside the medulla there is a dense network of reticular fibers that adequately support the loose parenchyma of the lymphatic organ. In normal conditions, the blood vessels of the medulla have a reduced volume and are hardly visible.
Lymphocytes penetrate the lymph node through two ways: through the afferent lymph vessels, if present in lymph, or through high endothelial venules (HEV), if present in blood.
The lymph penetrates the lymph node through lymph vessels (pre-nodal lymphatic collectors) crossing the capsule. Then, it spills in the subcapsular sinus and crosses the medullary sinuses. Finally, it reaches the lymph vessel (post-nodal lymphatic collector) and leaves the organ through the hilum. The lymph that reaches the lymph node can also directly cross the parenchyma to merge into the post-nodal lymphatic collector. This type of lymph flow through the lymph node allows cells that have absorbed the antigen (e.g. dendritic cells) or free microbes to stop inside the lymph node and enable an immune response.
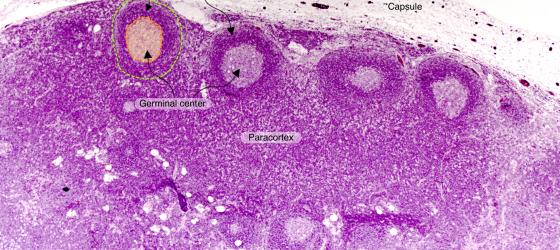
Figure C126A. Human lymph node. In the cortex of the lymph node, you can see several follicles. In the center of the follicle, there is a lighter zone, the germinal center, rich in B lymphocytes, surrounded by a darker external zone, the mantle, rich in T lymphocytes. H&E X25
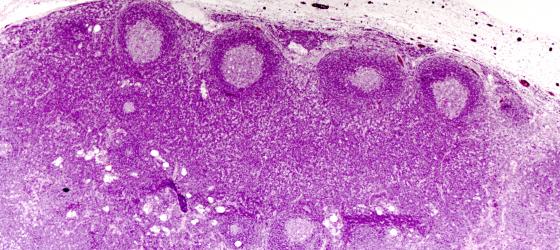
Figure C126B. Human lymph node. In the cortex of the lymph node, you can see several follicles. In the center of the follicle, there is a lighter zone, the germinal center, rich in B lymphocytes, surrounded by a darker external zone, the mantle, rich in T lymphocytes. H&E X25
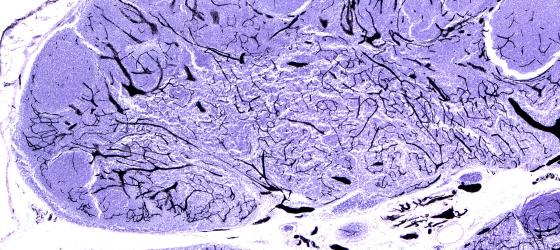
Figure C127. Mouse lymph node. Vital staining with India ink that highlights, in black, the vascular network of the organ. India ink X63
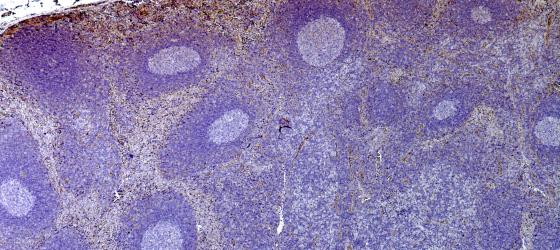
Figure C128. Human lymph node. Cortex of a lymph node cut with a cryostat. You cannot see the medulla because the lymph node was cut on the surface. DAB- Ematossilin X25
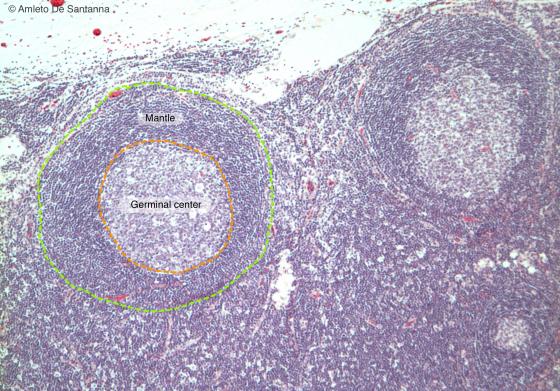
Figure C129. Human lymph node at higher magnification. You can see two follicles in the cortex of a lymph node. In the center of the follicle, there is a lighter zone, the germinal center, surrounded by a darker external zone, the mantle. H&E X63
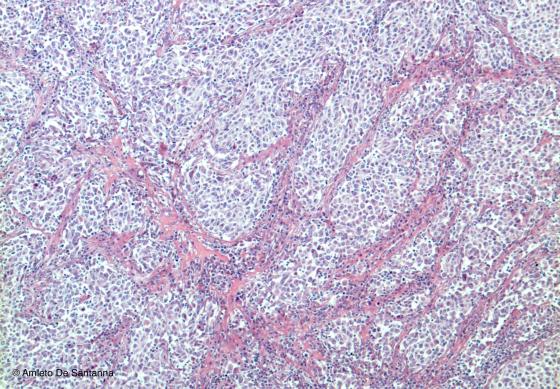
Figure C130. Human lymph node. The medulla is characterized by medullary cords (lighter zones) and by medullary sinuses, stained deep pink. H&E X40
Mucosa-associated lymphatic tissue (MALT)
Tonsils, the Peyer's patches and the vermiform appendix belong to the MALT. The lymphatic nodules present in these formations have an organization and a particular, characteristic stable structure: they are an integrated component of the organs they are immersed in. Moreover, they have no connective capsule to mark their border (except for the palatine tonsil hemicapsule), but present a dense meshwork to support them, in its typical ovoid shape. The mucosa-associated lymphatic tissue is organized in follicles that can be either primary or secondary, depending on the presence of a germinal center. Microscopically, tonsils are characterized, other than for the several follicles, for the presence, on their free margin, of a stratified squamous epithelium, typical of the first section of the digestive system (i.e. the palatine tonsils) or of a pseudostratified ciliated epithelium, typical of the respiratory system (i.e. the pharyngeal tonsils). Deeply inside the epithelium, there is a subepithelial zone, interposed between the epithelium and the follicles. Conversely, the vermiform appendix is characterized by a group of lymphatic follicles surrounding a lumen, an extension of the intestinal lumen, covered by a columnar epithelium and by the presence of simple tubular glands.
Moreover, all connective tissues, especially the loose ones underneath the epithelia, may contain accumulation of lymphocytes at different developmental stages. Such accumulations are recognizable due to the presence of small, highly colored cells, the lymphocytes, and their volume can vary significantly depending on the level of inflammation of the tissue. These accumulations neither present follicles nor stromal architecture nor, in general, a specific spatial organization.
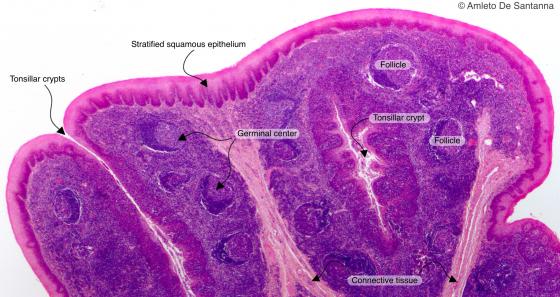
Figure C131A. Human palatine tonsil. Low magnification micrograph of a palatine tonsil where you can see, under a stratified squamous epithelium, the lymphoid tissue organized into follicles. The presence of stratified squamous epithelium let us to distinguish the palatine tonsils from the pharyngeal tonsils. H&E X12.5
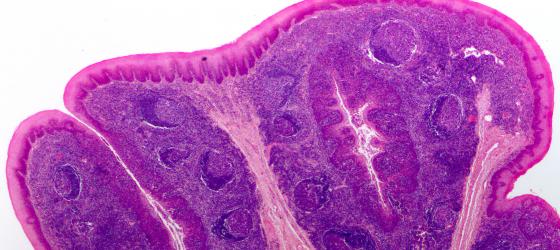
Figure C131B. Human palatine tonsil. Low magnification micrograph of a palatine tonsil where you can see, under a stratified squamous epithelium, the lymphoid tissue organized into follicles. The presence of stratified squamous epithelium let us to distinguish the palatine tonsils from the pharyngeal tonsils. H&E X12.5
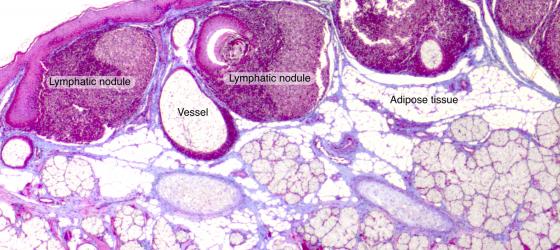
Figure C132. Human lingual tonsil. Lingual tonsil where you can see, under a stratified squamous epithelium, the lymphoid tissue organized into follicles. Mallory-Azan X25
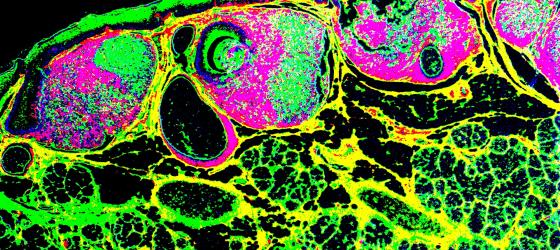
Figure C133. Human lingual tonsil. Digitally processed micrograph to highlight the lymphatic nodules. X25
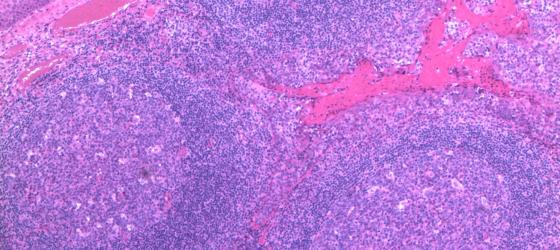
Figure C134. Human palatine tonsil. Under the stratified squamous epithelium, you can see three lymphatic nodules, each with a germinal center in the middle. H&E X40
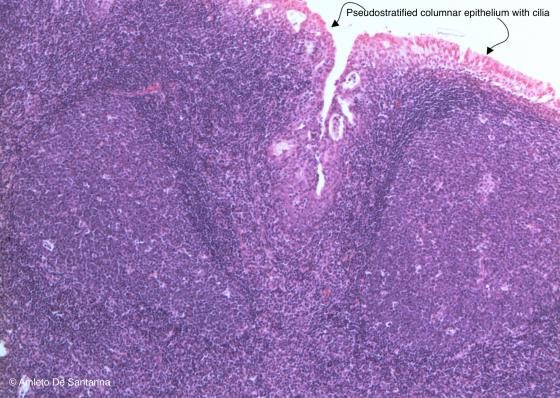
Figure C135. Human pharyngeal tonsil. You can distinguish the pharyngeal tonsil from the palatine and lingual tonsils because the tissue is lined by a ciliated pseudostratified epithelium, typical of the rhinopharynx. H&E X40
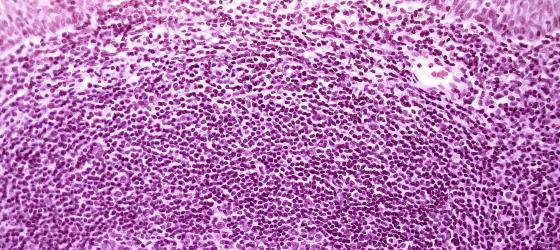
Figure C136. Human pharyngeal tonsil. Human pharyngeal tonsil at higher magnification to highlight the ciliated pseudostratified epithelium of the rhinopharynx. H&E X200
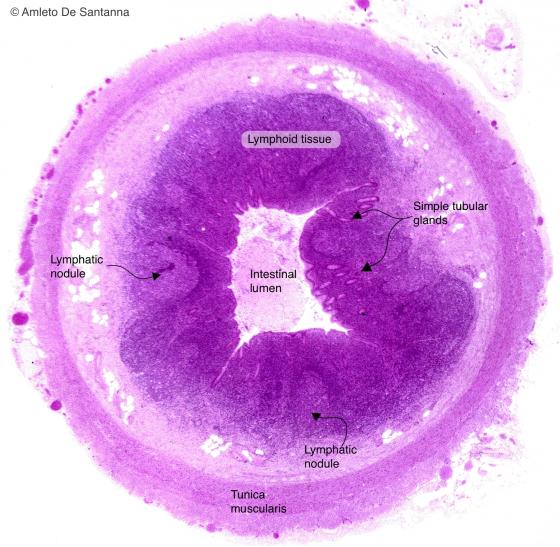
Figure C137A. Human vermiform appendix. Transverse section. This low magnification micrograph allows to esteem the organization of the appendix. H&E X12.5
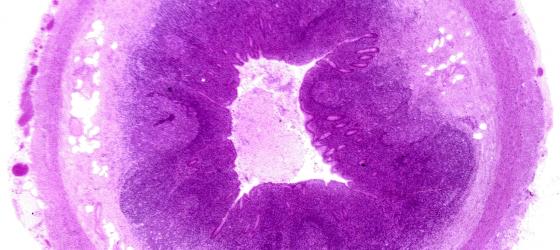
Figure C137B. Human vermiform appendix. Transverse section. This low magnification micrograph allows to esteem the organization of the appendix. H&E X12.5
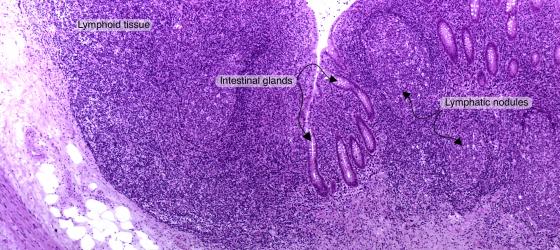
Figure C138A. Human vermiform appendix. You can see the intestinal glands, the simple columnar epithelium with goblet cells and a lymphatic follicle. H&E X40
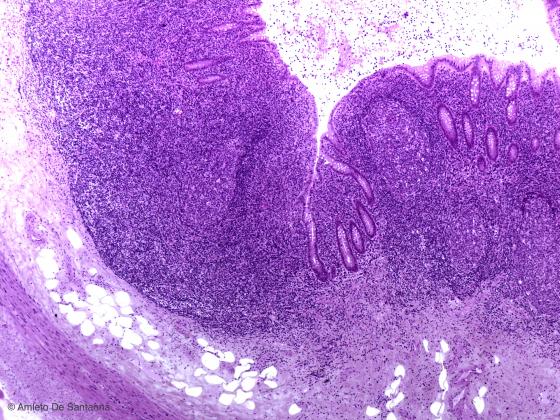
Figure C138B. Human vermiform appendix. You can see the intestinal glands, the simple columnar epithelium with goblet cells and a lymphatic follicle. H&E X40
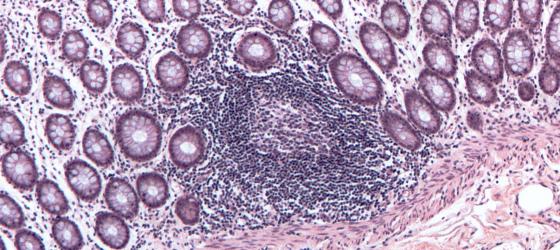
Figure C139. Human colon. Lymphatic nodules, transverse section. H&E X40
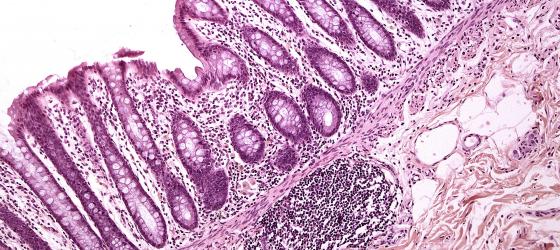
Figure C140. Human colon. Lymphatic nodules, transverse section. H&E X100
Lymphocytic infiltrations
In pathologic conditions, it is possible to highlight the presence of lymphocytic infiltration caused by inflammatory reaction inside the organs.
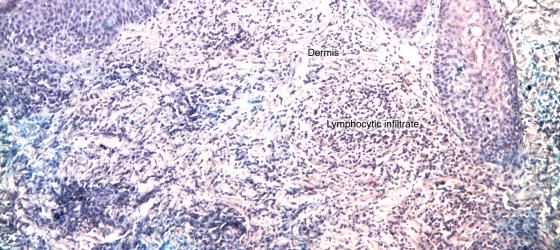
Figure C141A. Human skin. Lymphocyte infiltrate in a patient with lupus erythematosus sistemicus. Alcian Blue-Ematossilin X100
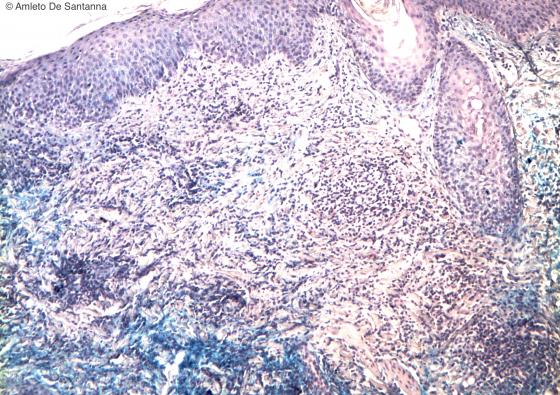
Figure C141B. Human skin. Lymphocyte infiltrate in a patient with lupus erythematosus sistemicus. Alcian Blue-Ematossilin X100
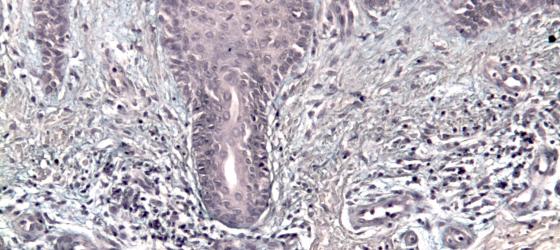
Figure C142. Human skin. Lymphocyte infiltrate in the dermis of a patient with psoriasis. Alcian Blue-Ematossilin X100
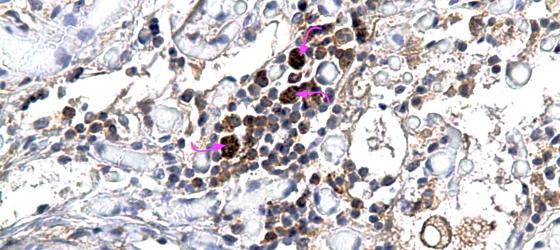
Figure C143. Dolphin lung at high magnification. Lymphocyte infiltrate and macrophages in a lung infection. Both lymphocytes and macrophages (arrows) are positive for the protein Bcl-2 (brown precipitate), a protein that promotes cell surviving by inhibiting apoptosis. DAB-Ematossilin X200
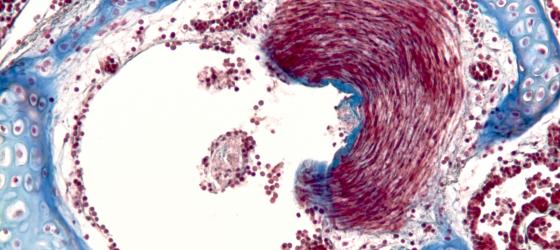
Figure C144. Dolphin lung. Strong lymphocyte infiltrate in a bronchus. Mallory-Azan X100
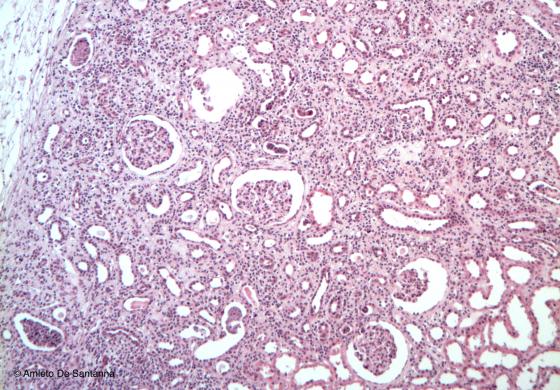
Figure C145. Mouse kidney. Strong lymphocyte infiltrate in the cortical zone and, in particular, in the glomeruli. H&E X40
Blood
Blood is a typical red fluid that circulates in a closed system of channels: the blood vessels. It derives from the mesenchyma and is composed of a corpuscular part (erythrocytes, leukocytes and platelets) and a liquid part (plasma). Its main function is to transport oxygen to the cells inside the tissues, other than transporting hormones. Leukocytes, also known as white blood cells, are common in the blood and in connective tissues, where they have a more ameboid shape rather than a more or less round shape. Only erythrocytes and platelets carry out their functions entirely inside the vascular system.
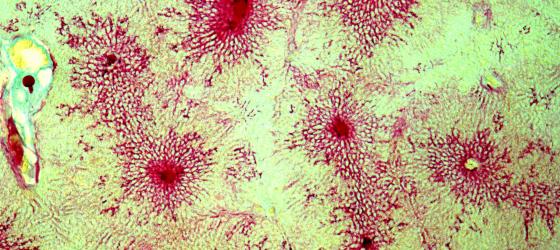
Figure C146. Mouse liver. Injection of vital stain that highlights the vascular architecture of the liver parenchyma. X25
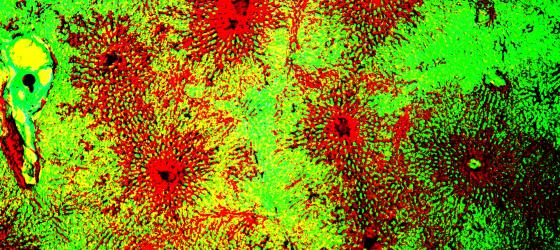
Figure C147. Mouse liver. Digitally processed micrograph. Injection of vital stain that highlights the vascular architecture of the liver parenchyma. X25
Erythrocytes
These are small cells rich in hemoglobin, the substance from which they get their red color. Their main function is to bind oxygen inside the lungs and transport it to the various tissues. During maturation in the bone marrow, they lose DNA-dependent properties and the nucleus regresses until it disappears. In fish, reptile and bird erythrocytes, the nucleus is still present. The reduced size of red blood cells allows them to cross the most peripheral capillaries, while their typical biconcave shape makes sure that, size being equal, they have a greater exchange surface.
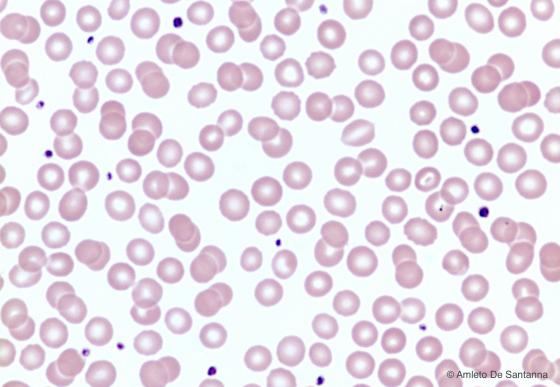
Figure C148. Human peripheral blood smear. Erythrocytes are stained pink. The lighter staining of the central part of the erythrocyte is due to their biconcave conformation typical of these cells. The darker cell fragments are platelets. H&E X100
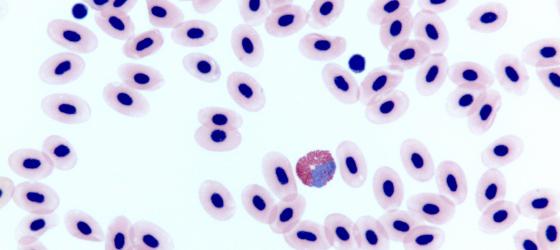
Figure C149. Frog blood smear. You can see that the erythrocytes have a nucleus and, in the center of the micrograph, an eosinophil. H&E X400
Platelets
Platelets are created inside the bone marrow, because of the budding of small parts of large cell cytoplasm, called megakaryocytes. Therefore, they are small, round or elongated corpuscles without a nucleus and microscopically visible only at high magnification, because their size is reduced compared to other blood elements, including erythrocytes. Platelets take part in the coagulation process, which is essential to reduce haemorrhages.
Leukocytes
Their nuclei are highly visible. When in the blood, they have a round shape, while in the connective tissue they take on an ameboid aspect. There are five types of leukocytes, classified depending on the presence and amount of cytoplasmic granules (called granular or agranular leukocytes) and on the shape of the nuclei (monomorphic nuclear leukocytes and polymorphonuclear leukocytes).
Granular leukocytes are classified depending on the staining characteristics of the cytoplasmic granules: basophilic, eosinophilic and neutrophilic.
Agranular leukocytes are divided, according to their size and shape of their nucleus, in lymphocytes and monocytes.
Neutrophils
These are the most numerous leukocytes, around the 50-70% of the total leukocytes. With a light microscope, they can be distinguished because of the shape of their nucleus, which presents three or more lobes, connected by thin chromatin bridges. In addition, bilobed nuclei have been detected, but they are very rare (at least in the blood). The number of lobes depends on the age of the cell: the more lobes it presents, the older the cell is. From these data, the level of regenerative capacity of the hemopoietic tissue may be easily deduced. In approximately 3% of these cells, a small, nuclear, drumstick-shaped appendage can be seen. This appendage is called Barr body (inactive X chromosome), an indicator of the female gender. Despite being clearly visible, the neutrophilic granules are generally poorly stained, hence their name. Neutrophils have phagocytic capabilities and contribute to protect the organism against bacterial infections. To do so, they migrate towards the endothelial cells and penetrate the connective tissue where they may carry out their function at their best. During bacterial infections, these leukocytes are produced in larger amounts.
Eosinophils
They are easy to recognize because of their specific granules, intensely stained with eosin and acidic staining methods in general. These cells always present a bilobed nucleus, whose lobes are connected by a thin isthmus. Generally, they represent 2-4% of blood circulating leukocytes, but they increase remarkably in case of allergy, parasite attack, inflammation and neoplastic disease.
Basophils
Si riconoscono per la forte granulazione basofila del citoplasma che, con l’ematossilina, assume un acceso colore violetto che, di conseguenza, tende a nascondere il nucleo a forma di U o di S. Sono circa lo 0,5-1% dei leucociti circolanti. I grossi granuli basofili, contenenti eparina e istamina, sono ricchi di lisosomi che, a differenza di eosinofili e neutrofili, rilasciano il loro contenuto all’esterno e non nei vacuoli fagocitati. Intervengono sia nelle reazioni infiammatorie classiche sia nelle reazioni allergiche.
Lymphocytes
Most of the blood circulating lymphocytes are small round cells. They are highly pigmented and present a nucleus filling a great part of the cell, thus leaving only a thin peripheral ring to the cytoplasm. These cells represent 20-40% of the blood circulating leukocytes. Morphologically, T lymphocytes, B lymphocytes and NK lymphocytes cannot be distinguished from one another.
Monocytes
Monocytes represent 3-8% of blood circulating leukocytes. They are large, their nucleus is evident and its lobes are more numerous depending on the age, so that their look from that of a kidney to a horseshoe. Although the cytoplasm is rich in primary lysosomes, it does not present granules, which might be visible with a light microscope. They pass through the blood and migrate towards the connective tissue where they turn into either macrophages or dendritic cells.
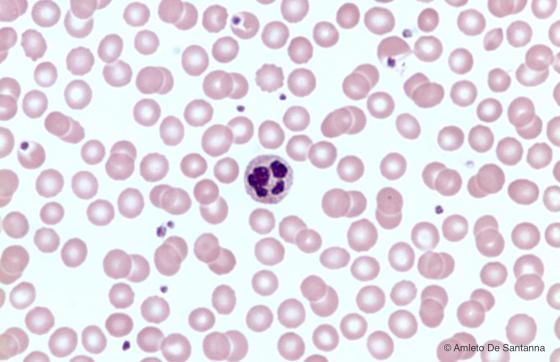
Figure C150. Human peripheral blood smear. In the center of the micrograph, there is a granular leucocyte (neutrophil) with a polylobed nucleus. H&E X400
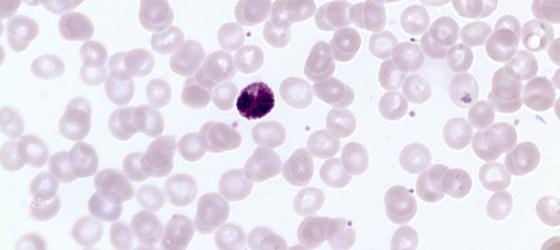
Figure C151. Human peripheral blood smear. Basophilic granular leucocyte (basophil) identifiable by the deep purple staining (by hemalum) of the rough cytoplasmic granulation. The nucleus is hidden by the basophilic granulation. H&E X400
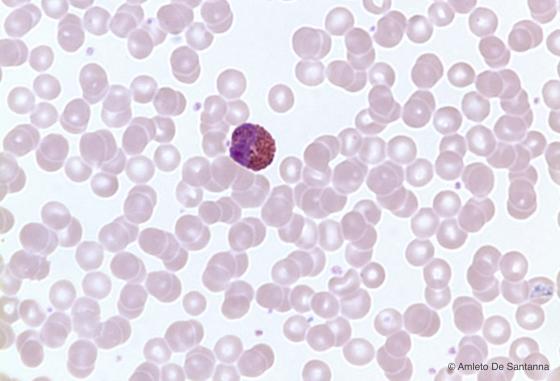
Figure C152. Human peripheral blood smear. Eosinophilic granular leucocyte (eosinophil) with an obvious deep pink granulation and a nucleus, generally bilobed. H&E X400
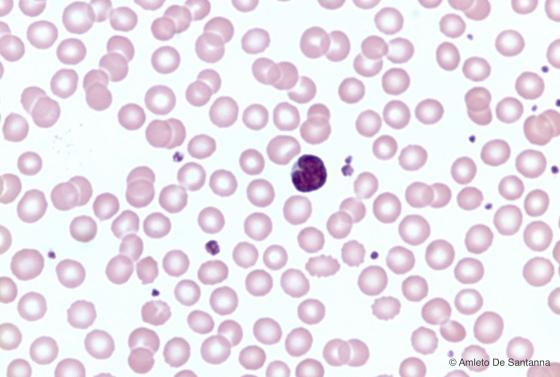
Figure C153. Human peripheral blood smear. Agranular leucocyte (monocyte) with a kidney shaped nucleus and cytoplasm without granulations. H&E X400
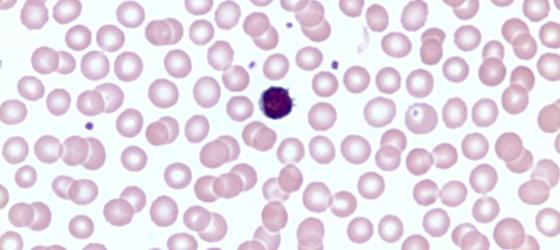
Figure C154. Human peripheral blood smear. Small agranular leucocyte (lymphocyte) with a roundish large nucleus without indentations that occupies almost the 90% of the volume of the cell, leaving just a narrow rim of cytoplasm. H&E X400
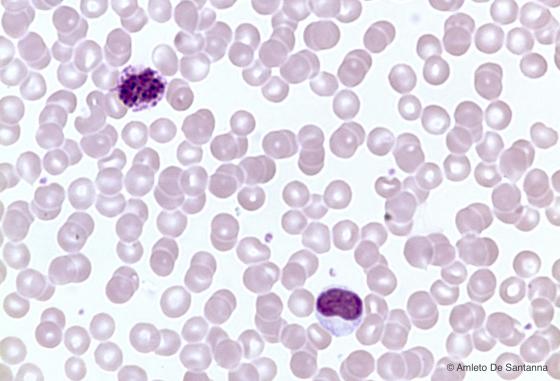
Figure C155. Human peripheral blood smear. In the upper part of the micrograph, you can see a basophilic granulocyte, in the lower part a monocyte. H&E X400
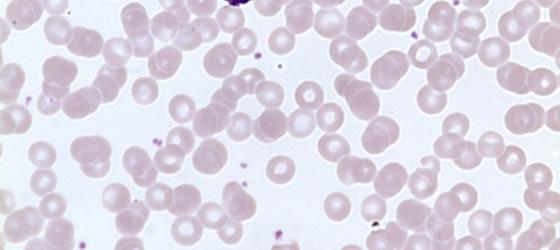
Figure C156. Human peripheral blood smear. In the upper part of the micrograph, you can see a basophilic granulocyte, in the lower part a lymphocyte. H&E X400